THERAPY OF UVEAL MELANOMA
A REVIEW
Authors:
Š. Rusňák 1; L. Hecová 1; Z. Kasl 1; M. Sobotová 1; L. Hauer 2
Authors place of work:
Oční klinika, Fakultní nemocnice v Plzni
1; Stomatologická klinika, Fakultní nemocnice v Plzni
2
Published in the journal:
Čes. a slov. Oftal., 77, 2021, No. 1, p. 3-15
Category:
Summary
The aim of intraocular melanoma therapy is to achieve local tumor control, reduce the risk of metastasis development, preserve the eyeball and possibly the visual function of the eye. The choice of therapeutic approach requires a comprehensive view and individual approach to each patient with uveal melanoma. Factors considered include local finding (location, tumor size and shape, tumor activity, central visual acuity, intraocular complications), age and the patient‘s overall physical and psychological condition, as well as the patient‘s wishes.
The most widely used method of uveal melanoma treatment is radiotherapy. The effect of radiation is caused by the absorption of ionizing radiation energy, the effect of radiation on the cell is manifested by cell death (depletion), or by a cytogenetic information change (mutation). Brachytherapy uses scleral applicators with radionuclide - ruthenium (Ru-106) applicators dominate in Europe and iodine (I-125) applicators in the USA. In external radiotherapy, the source of ionizing radiation is outside the patient‘s body. Both stereotactic radiosurgery and fractionated stereotactic radiotherapy are used. In the Czech Republic, treatment is carried out using Leksell gamma knife or CyberKnife, while proton therapy dominates in the world. The development of serious radiation complications (radiation retinopathy, neuropathy, neovascular glaucoma, toxic tumor syndrome, etc.) should be considered.
Surgical therapy involves a variety of invasive procedures. Iridectomy is performed for iris melanoma. Anteriorly located choroidal melanomas and / or ciliary body melanomas can be resolved by transscleral resection (exoresection). For posterior choroidal melanomas, a combination of external tumor irradiation with pars plana vitrectomy is used.
Enucleation is a method of choice in advanced tumors that cannot be effectively irradiated. Orbital exenteration is indicated in advanced tumors with extrabulbar spread or in relapsed tumor after previous enucleation.
Keywords:
radiotherapy – brachytherapy – uveal melanoma – teleradiotherapy – endoressection – exoressection
Therapy of uveal melanoma
The aim of intraocular melanoma therapy is to achieve local control over the tumour and reduce the risk of metastasis of the pathology, while at the same time efforts predominate to preserve the eyeball and also the visual function of the eye. The choice of therapeutic approach for patients with uveal melanoma requires a comprehensive overview and an individual approach to each patient. The factors taken into account include the local finding itself (localisation, size and shape of tumour, signs of activity of tumour, visual acuity, presence of intraocular complication), above all the age and overall physical and psychological condition of the patient, and also the patient’s wishes [1].
The gold standard in the treatment of uveal melanoma is radiotherapy – primarily brachytherapy, in the case of larger tumours teleradiotherapy, if applicable in combination with resection of the tumour. In recent years there has been a change of the therapeutic approach above all in the case of small melanocytic lesions. The risk of metastasis of uveal melanoa is dependent above all on the cytogenetic and histopathological characteristics of the tumour, but in the case of very small melanomas (with a tumour height of up to 3 mm) the risk of generalised pathology is low, including in the cases of a risk histopathological and cytogenetic finding [2,3]. It has also been determined that the histopathological and cytogenetic characteristics of certain originally prognostically favourable lesions change over the course of time, and become high risk lesions with regard to the probability of metastasis of the disease [4]. As a result there is an increasing tendency in favour of precisely diagnosing and treating also small lesions, which in the past were only observed. Biopsy techniques, which enable timely diagnosis of small or clinically ambiguous melanocytic lesions, are also increasing in importance [5,6].
Regular observation
Regular observation is used in the case of small suspect lesions without signs of activity and without evidence of growth (according to photo documentation, autofluorescence, ultrasonic examination or optical coherence tomography (OCT)), or for older or seriously ill patients without local complication of the tumour.
Laser photocoagulation
The technique of photocoagulation of uveal melanoma using an argon or krypton laser is no longer used today. In the past it was used rather exceptionally in the case of choroidal melanomas with small prominence, localised in the region of the posterior pole of the eye [7].
Transpupillary thermotherapy
Transpupillary thermotherapy (TTT) is performed by means of a diode laser with a wavelength of 810 nm. This method generates local hyperthermia in the tissue to a depth of 4 mm [8,9,10]. Previously TTT was used as monotherapy for small choroidal juxtapapillary or juxtafoveal tumours, but is no longer recommended as a primary method today. At present TTT is applied primarily in combination with radiotherapy (e.g. for treatment of the central periphery of juxtapapillary lesions) [11]. A complication of this method is outages in the visual field and maculopathy.
Photodynamic therapy
Photodynamic therapy (PDT) uses an intravenously administered photosensitive substance, which is selectively uptaken in the newly formed blood vessels. Subsequent irradiation of these blood vessels causes their thrombotisation and the destruction of the vascular endothelium by oxygen radicals, which are released upon a photochemical reaction [12]. Today it is no longer used as a primary therapy in the treatment of uveal melanoma.
Radiotherapy
Radiotherapy (i.e. therapy by ionising radiation) is the gold standard in the treatment of uveal melanoma (especially choroidal melanoma). Both local radiotherapy with the aid of scleral applicators with radionuclides (brachytherapy) and external radiotherapy (teleradiotherapy), in which the source of ionising radiation is outside the body of the patient, are used.
The actual effect of radiation on the living tissue is generated by the absorption of the energy of ionising radiation, in which excitation of atoms and molecules occurs and energy is transmitted to electrons. The transmission of energy in the living tissue is of a quantum character – in a homogeneously irradiated sample, areas with a higher number of ionisations and excitations can be found, as well as areas where no transmission of energy took place. In order to attain a biological effect, it is necessary for the radiation to target a certain sensitive structure, which in biological objects is most frequently a DNA molecule. Radiation changes of DNA are highly diverse, in which the most typical change is fragmentations of DNA – simple or dual, as well as damage to the bases or local denaturation of DNA. However, biological systems usually contain a high percentage of water, where a substantial proportion of radiation is absorbed. “Radiolysis” of water generates highly reactive products such as the hydroxyl radical OH, the hydrogen radical H or hydrogen peroxide molecules H2O2. All these products may enter further reactions and thereby initiate a so-called indirect effect of radiation.
The effect of radiation on the cell is manifested either by direct cell death – cellular depletion, or change of the cytogenetic information of the cell – mutation. Cell death may occur in the clinical phase – the interphase between two phases of division. This effect is conditioned by the high dose of radiation which leads to the general denaturation of the cellular structures. The second variant is death of the cell in the phase of division, in which cell damage is manifested by the inability of the cell to divide. This “mitotic catastrophe” occurs upon significantly higher doses, and is manifested most easily in the tissues characterised by rapid cell division – in addition to tumours for example haematopoietic tissue, the intestinal endothelium or a developing embryo.
The effect on the organism is manifested partially in the acute phase and partially through later damage. In the acute phase, upon full-body irradiation with a dose from 1 Gy, we observe acute radiation sickness, in which symptoms from disorders of haematopoiesis (1-6 Gy), the gastrointestinal tract (around 10 Gy) up to damage to the central nervous system (tens of Gy) predominate in the clinical picture. The most common local acute reaction is dermatitis, the degree of damage depends upon the dose (3 Gy and more), the type of equipment, size and localisation of the irradiated field. Another common local effect of irradiation is for example radiation inflammation of the nasopharynx following single-dose irradiation of the orbit with a damage threshold of approximately 5 Gy. Later damage as a consequence of ionising radiation is manifested e.g. in changes to the lens (a single dose of around 1 Gy is cataractogenic) or chronic post-radiation inflammation of the skin, which represents a challenging problem especially in the region of the eyelids.
The destructive effect of ionising radiation on the tissue of the melanoma is desirable: the tumour cells succumb to vacuolation of cytoplasm, rupture of the nuclei, they are infiltrated by neutrophils, lymphocytes and plasma cells. The debris of necrotic cells, as with cells in the stage of apoptosis, is phagocyte macrophages. Depletion of endothelial cells leads to vascular infiltration, and eventually to occlusion of the vascular lumen, and a reparation process appears – fibrosis [13] (Fig. 1). The destructive effect of radiation inevitably afflicts also collateral structures, which is an undesirable effect that with regard to the intimate proximity of practically all the surrounding tissues may induce the development of changes leading to loss of the eye.
Brachytherapy
Brachytherapy is the most frequently used method of treatment for uveal melanoma [14]. The successful use of brachytherapy in the treatment of choroidal melanoma was first mentioned in 1915 by Deutschmann in Hamburg, who used mesotherium in the treatment of a patient with a tumour in one eye [15]. In 1929, Moore implanted a radon nugget into the eye of a patient with choroidal sarcoma in the sole functioning eye [16]. This technique was developed by Stallard in the 1930s in the treatment of retinoblastoma – Stallard coated a radon nugget in wax, placed it on the surface of the eye and later removed it [17].
In brachytherapy scleral applicators are used, containing various types of radioactive elements. After localisation of the tumour by translumination and indirect ophthalmoscopy, the applicators are sutured to the sclera externally above the place of the base of the tumour under general anaesthesia, and are left here for a sufficient period for a dose of usually 80-100 Gy (according to the type of applicator) to be attained to the apex of the tumour, after which the applicator is removed from the eye. For the choice of applicator and calculation of the time of brachytherapy, it is essential to ensure the precise determination of the size of the tumour (maximum height and size of base of tumour) and the current activity of the used radiator. Today applicators using radionuclides Ru-106 and I-125 predominate. Lesions with the base of the tumour of up to 20 mm and with a prominence of up to 10 mm are indicated for brachytherapy in the case of iodine applicators, or of up to 7 mm in the case of ruthenium applicators. Regression of the tumour begins 1-2 months after therapy and persists for a number of years. The success rate of brachytherapy of uveal melanomas (i.e. attainment of local control over the tumour and preservation of the eyeball) fluctuates according to the type of used applicator and radionuclide, size and localisation of the tumour and experience of the operating surgeon [18].
Ruthenium 106
The most frequently used radioisotope in brachytherapy in Europe is the β-radiator Ru-106. Brachytherapy with the use of Ru-106 was first presented by the German ophthalmologist Peter Lommatzsch in the 1960s [19]. Ocular applicators of Ru-106 contain the radionuclide ruthenium 106 in long-term balance with the subsidiary nuclide rhodium 106. The applicators emit predominantly beta radiation with a small admixture of gamma radiation. The half-life constitutes 373.6 days, and the nominal activity of the source is 4–31 MBq. T emitted beta particles have energy of 2.12 MeV. The usual application to the apex of the tumour is 100 Gy. The applicators are manufactured commercially in various sizes (average 11.6–25.4 mm) and shapes (rounded, with hole for the optic nerve or moulded for use in the region of the limbus). The core of the ruthenium applicator is composed of a 0.2 mm thick layer of aluminium covered by Ru-106/Rh-106, and is stored in a silver cover which functions as a radiation shield (absorbing approximately 95 % of beta radiation). The advantage of ruthenium applicators is the minimal radiation burden for the surgical team – an acrylate or silver dummy applicator is first of all sutured to the sclera after localisation, after which the actual ruthenium applicator is then placed on the prepared sutures. The applicator is stored and sterilised in a stainless steel container with aluminium lining (Fig. 2).
Brachytherapy of uveal melanoma with the use of applicators with Ru-106 provides excellent local control (according to various authors 73–98 % 5 years after treatment) with a low degree of recurrence of tumours in the case of tumours with a maximum height of up to 7 mm [20,21,22,23].
Iodine 125
In the United States of America, the most widely used isotope in the treatment of uveal melanoma has been the radioisotope I-125, emitting γ-radiation. In comparison with the previously used applicator with Co-60, in the case of the isotope I-125 there is a comparable penetration to a depth of 10 mm, but at the same time with pronouncedly lower affliction of the adjacent healthy tissues. Thanks to easier shielding of radiation, this type of applicator places a lower radiation burden on both the patient and the surgical team [24,25].
During treatment, a reusable gold cover of the applicator is used in combination with a single-use silicone insert, individually filled with capsules with the radioisotope I-125, which is fixed to the cover by means of a number of drops of adhesive silicone. Applicators of various sizes are available (usually with a diameter of 12–20 mm). The silicone inserts have holes into which titanium capsules with I-125 are inserted. The radioisotope I-125 has a half-life of 59.41 days. It breaks down after interception of the electron, and during its breakdown emits γ-radiation and X-rays, the emitted photons have energy of up to 35 keV. The emitted electrons are absorbed by the titanium capsule in which the radioisotope I-125 is stored.
Other radionuclides
Other types of radionuclides are also used to a lesser degree in brachytherapy of uveal melanoma. In the 1960s and 70s these included for example radon – a golden nugget filled with radon gas was evenly placed throughout the perimeter of a circular applicator, which was sutured to the exposed sclera in the place of the tumour, and with regard to the short half-life of radon (3.8 days) was left permanently on the eye in the case of good tolerance [14,26]. From the 1960s circular, crescent-shaped or semicircular applicators with cobalt 60 (Co-60) were widely used, in which they were sutured to the sclera above the base of the tumour, with an overhang of 1 mm over the edge of the tumour. The limited long-term effect of the treatment and the significant adverse side effects of radiation caused by the high energy which could not be shielded on the exterior surface led to the abandonment of the use of cobalt applicators in the second half of the 1980s, and their replacement with low-energy brachytherapy [27,28,29]. Since the end of the 1980s, applicators have been available using palladium 103 (Pd-103), emitting photons with low energy (on average 21 keV), which have better absorption by the tissue tumour than I-125 photons [30]. Strontium (St-90) emits high-energy β-radiation with good penetration to a depth of 4 mm, in the case of larger lesions it is necessary to repeat brachytherapy with this type of applicator [31]. Other elements which can be used in the therapy of uveal melanoma are e.g. iridium (Ir-192) or gold (Au-198).
Teleradiotherapy (external radiotherapy)
In comparison with surgical treatment or brachytherapy, stereotactic radiosurgery and fractionated stereotactic radiotherapy of uveal melanoma represents a less invasive therapeutic modality [32]. For safe and effective treatment using teleradiotherapy it is necessary on one hand to ensure perfect imaging of the lesion being treated, and on the other correct choice of irradiation method, with precise targeting and minimisation of damage to the surrounding tissue. In the Czech Republic the most widely used methods are Leksell gamma knife (LGK) and the cybernetic knife CyberKnife, in Slovakia treatment using a LINAC stereotactic linear accelerator is available, while worldwide proton therapy dominates in the treatment of uveal melanoma.
Irradiation of the tumour can be performed in a single session or in fractionated form. The greatest benefit of single-dose irradiation is greater patient comfort. The advantage of fractionated irradiation is the reduction of the radiation burden on healthy tissues and subsequent complications in maintaining good control over the tumour [33]. The frequency and severity of complications can also be reduced by reducing the used radiation dose [33,34,35,36].
Leksell gamma knife
The history of the invention and development of the gamma knife is linked with the Swedish scientist and neurosurgeon Lars Leksell. The first construction Gamma Unit I was launched into operation in 1968, and was focused on indications in the field of functional neurosurgery [37]. In the Czech Republic, Leksell gamma knife has been available since 1992 in the Na Homolce Hospital in Prague (Department of Stereotactic and Radiation Neurosurgery).
Leksell gamma knife uses ionising radiation in the form of photons. It concerns isocentrically focused gamma radiation from a large number of radioactive sources. Photons are generated on the basis of radioactive conversion of the radionuclides 60Co to 60Ni. In the monoenergetic beam an exponential reduction of the number of photons takes place, with increasing depth of penetration into the given material. The resulting depth dose curve depends on the primary spectrum of the photon beam, its collimation, the size of the beam and material. The dose increases initially in the direction from the surface to its maximum value, which is characterised always by a certain depth for the given energy of the photon beam, geometric layout and absorption material. The dose then decreases exponentially with the increasing depth. In order to obtain high absorbed doses in the target lesion while at the same time minimising the dose into the surrounding healthy tissue, it is necessary to use a large number of collimated isocentric beams, specifically beams intersecting in a single point. The resulting dose profile from all the sources in this volume then represents the course of the dose, in which we obtain the maximum absorbed dose in the place of the target lesion, where all the beams are projected, at the same time as a sharp reduction of the dose into the surrounding area [38].
Leksell gamma knife has three main components, i.e. radiation units with a radiation source of 60Co with 4 or 3 collimator helmets, a stereotactic instrument and the planning system Leksell GammaPlan.
Before the actual radiotherapy using Leksell gamma knife, current imaging diagnosis is performed (MR or CT), indicating the lesion on the basis of which the irradiation plan is compiled. Montage of the stereotactic frame is then performed, which can be fixed either invasively (with the aid of 4 aluminium screws) or non-invasively (with the aid of a soft mask placed on the face in combination with a sensor placed on the tip of the nose) to the patient’s skull, which ensures a constant position of the head structures.
The irradiation plan is compiled in such a manner as to guarantee the best distribution of the dose to the target area of irradiation, and at the same time to ensure maximum protection of the surrounding tissues and organs. In planning, the prescribed isodose is selected usually at a value of 50 %. The prescribed isodose should cover at least 95 % of the target volume, and the dose for this isodose is selected with regard to the pathology of the tumour, the volume of the irradiated lesion, the adjacent functionally significant structures and if applicable also previous irradiation [38].
Pathologies treated with the aid of Leksell gamma knife include benign and malignant tumours (e.g. meningiomas, adenomas of the pituitary gland, vestibular schwannomas, metastases in the brain and certain gliomas), vascular manifestations, functional pathologies and ocular pathologies [38].
Leksell gamma knife has been used in the treatment of uveal melanoma since the 1990s [32]. With regard to the stereotactic frame, the eyes are in excentric localisation, and as a result the patient usually lies on the abdomen during irradiation. For the safe performance of the treatment it is necessary to ensure a immobilisation of the irradiated eye – in retrobulbar anaesthesia 2 sutures are fixed to the stereotactic frame in the place of the ligament of the medial and lateral rectus muscles of the eyeball, and a specially shaped tub filled with sonographic gel is then applied to the eye. Immobilisation of the eyeball for a limited period can be performed also by simple retrobulbar application of a local anaesthetic [39], or the patient may fix the eye actively, e.g. by gaze at a point source of light [40]. The actual irradiation of uveal melanoma generally lasts for tens of minutes and is performed in a single session, usually in a dose of 20–50 Gy in 50 % isodose [41,42,43,44,45].
CyberKnife
The cybernetic knife was developed in 1994 by professor John Adler and his team from Stanford University. In 1999 CyberKnife obtained approval from the United States Food and Drug Delivery Administration (FDA) for radiation therapy of tumours of the head and neck, and in 2001 for radiation therapy of extracranial areas. Since 2010 CyberKnife has been in operation at the University Hospital in Ostrava, in 2020 a further CyberKnife is due to be launched into operation at the Central Military Hospital.
CyberKnife is a linear accelerator specially adapted for stereotactic radiotherapy and radiosurgery, which enables single-dose or fractionated precise irradiation of intracranial and extracranial tumours. Photons with energy of 6MV are artificially created by the radiation source. The linear accelerator generates very thin radiation beams which are emitted from 1200 different positions and intersect in the place of the target being irradiated. The beams themselves have a very small proportion in the total dose of radiation, and as a result the healthy tissue which they pass through is not damaged. This method enables the irradiation of the target tissue with a high degree of precision and minimal toxicity for the surrounding tissues [46,47]. The linear accelerator is located at the end of a robotic arm, which is able to move around the recumbent patient within a range of 358°. During the course of irradiation the patient lies on a robotically controlled table, which is adjustable in 6 directions within a range of 10°. In addition to the high precision of targeting the lesion, the CyberKnife has the ability to observe movements of the patient in real time (by 2 X-ray detectors located on the ceiling of the radiation room), and adapt irradiation accordingly. The irradiation targets, which move in connection with the patient’s breathing, are monitored with the aid of a Synchrony system.
Before the planned and actual stereotactic radiotherapy or radiosurgery, a stereotactic mask is first of all created for the patient, which limits movement of the head and neck during irradiation. This concerns non-invasive fixation by means of a thermoplastic mask, shaped according to the patient’s face. After application to the patient’s face, the mask is secured to a semicircular frame [48]. A planning CT, MR or PET examination is subsequently conducted for the purpose of precise determination of the size, shape and localisation of the tumour. The doctor then demarcates the size of the area to be irradiated using CyberKnife software, designates the radiation dose and identifies the structures where the radiation is to be minimised, and compiles an irradiation plan. The cybernetic knife is capable of targeting a lesion in immobile targets with a precision of 0.5 mm, in mobile targets up to 2 mm [46], and can be used to irradiate a lesion with a size of up to 5 cm and a maximum of 3 lesions [46,47,49].
CyberKnife is used for the treatment of intracranial and extracranial benign and malignant tumours (e.g. tumours of the head and neck, vertebrae, pancreas, liver and prostate). Within the framework of stereotactic radiosurgery it is possible also to treat trigeminal neuralgia. Intracranial and extracranial stereotactic therapy is used especially on patients who are unable to undergo surgical treatment due to the anatomical localisation of the tumour or in the case of inoperability due to internal causes [47,48,49].
In ophthalmology it is used in the treatment of medium- -sized and large uveal melanomas, both within the framework of stereotactic radiosurgery and in fractionated stereotactic radiotherapy [50,51]. Immobilisation of the eye is ensured by means of application of retrobulbar anaesthesia [52] or the placement of a flashing fixation light above the healthy eye and a camera recording eye movement above the eye afflicted by the tumour. For monitoring eye movement and better targeting of the uveal tumour it is possible to use a lens with implanted golden nuggets [52]. The optimum total radiation dose and schema of fractions are not yet unequivocally stipulated. Both single-dose and fractionated radiation in 3–7 sessions are used [50,53].
Linac
In Slovakia the stereotactic linear particle accelerator LINAC is also used in the treatment of uveal melanoma. This was first experimentally used in London in 1952, and since 1953 has been used in the treatment of human patients [54]. According to available studies, radiotherapy with a LINAC linear accelerator is effective in the case of small and medium-sized melanomas, in fractionated stereotactic radiotherapy the total dose is usually 50–70 Gy to 80 % of the isodose [55,56] in stereotactic radiosurgery a single dose of 25–35 Gy [57,58].
Proton therapy
In 1954 the first therapeutic experiments with proton radiation were conducted in the radiation laboratory in Berkeley and in 1957 in Uppsala, initially on pituitary tumours. In 1961 co-operation was commenced between the cyclotron laboratory in Harvard and the General Hospital in Massachusetts, with the aim of focusing on proton therapy. The proton therapy programme was improved and developed up to the closure of the cyclotron laboratory in 2002, and 9116 patients were treated here. In 1990 the first university proton centre was established at Loma Linda in California [59,60].
Radiotherapy by solid particles uses “hadrons” (heavy particles), which primarily include protons, neutrons and light ions. In clinical practice, positively charged particles of the nucleus of a hydrogen atom (protons) are used most frequently (up to 90 %), while carbon ions or helions are used less often. Proton radiation, similarly to photon radiation (X-rays and gamma-radiation) or electrons, is ranked among beams with low relative biological effectiveness. Protons are produced in a cyclotron by means of the removal of electrons from a hydrogen atom, and are subsequently accelerated by the cyclotron to a speed of approximately half the speed of light. They thereby acquire energy of up to 230 MeV, which enables the destruction of tumours to a depth of 30 cm. The protons are then directed by a strong magnetic field into a pencil beam, with a flow of more than 2 trillion protons per second and with a high degree of precision are transmitted to the base of the spatially displayed tumour. Upon deceleration in the tumour tissue there is a release of energy, ionisation, release of free radicals and damage to the DNA of the targeted cell [60]. The main advantage of proton therapy is that the proton beam transmits the greatest part of its energy in the phase of the Bragg peak, i.e. in the area shortly before the end of the flying range of the particle in the matter where the majority of energy is absorbed. In the healthy tissues before the tumour, the proton beam loses energy only minimally. Depending on the depth of the localisation of the tumour beneath the surface of the body, 70–80 % of the energy of the beam reaches the area of the tumour. Beyond the Bragg peak the radiation dose is zero. An advantage of proton therapy is therefore the minimal damage to the healthy tissue within the immediate vicinity of the tumour, the high dose within the area of the tumour and the possibility of irradiating also larger tumours. A relative disadvantage of proton therapy is the high price of treatment in comparison with conventional radiotherapy or other therapeutic procedures [61].
Proton therapy is used in the treatment of tumours of the CNS as primary radiotherapy (e.g. meningiomas in the region of the cranial base or juxtaspinal tumours) or as postoperative radiotherapy following incomplete resection or in the case of early recurrence of a tumour. Both fractionated proton therapy and proton stereotactic radiosurgery are used. Another area of indication is solid tumours in childhood age (e.g. medulloblastomas, craniopharyngimoas, inoperable gliomas, ependymomas), invasive and/or non-bordered tumours with a tendency to infiltrative growth, also e.g. tumours close to important anatomical structures (e.g. optic nerve, chiasma, eye, spinal cord or large jugular blood vessels), radioresistant tumours and tumours with a high risk of local recurrence, tumours of the paranasal sinuses, carcinomas of the thyroid gland, tumours of the lachrymal gland, carcinomas of the nasopharynx or oropharynx, and prostate cancers.
In the treatment of uveal melanomas, a proton beam with energy of 62–68 MeV is used [62,63]. In rare cases proton radiation is used also in the therapy of melanoma of the conjunctiva, retinoblastoma and haemangioma of the retina or choroid, or age-related muscular degeneration [64]. Before the actual radiotherapy takes place, localising tantalum markers are sutured to the sclera (after localisation of the tumour by translumination, indirect ophthalmoscopy or opto-electric navigation system), which define the boundary of the tumour [65,66]. During the procedure the precise position of the localising markers is recorded, as well as the size, shape and position of the tumour with regard to the optic nerve papilla, macula and localising markers [67]. In the case of melanoma of the iris, tantalum markers are not used [68]. A made-to-measure fixation mask with a special gum shield is subsequently constructed, and X-ray images of the patient’s head are taken in a therapeutic position with 4 possible gaze positions of the eye [63]. The obtained data is stored in interactive 3D planning software (e.g. Eyeplan software, Octopus software), which calculates the therapeutic parameters, including the angle of fixation, modulation of the proton beam or relative position of the localising markers with regard to the proton beam. Therapy takes place in fractionated form – depending on the local finding and experience of the proton centre in question this is usually 4–5 sessions on consecutive days, or over the course of 7–10 days. The irradiation during each session usually lasts for 30–60 seconds, in total a radiation dose of 60–70 CGE (Cobalt Grey Equivalent) is applied into the tumour [63,65,69].
The closest proton centres offering proton therapy of uveal melanoma are the BerlinProtonen centre at the Charité hospital in Berlin, the proton centre at the Paul Scherrer Institute (PSI) in Villigen, Switzerland, and the Cyclotron Centre Bronowice in Krakow, Poland.
Complications following radiotherapy of uveal melanoma
The effects of ionising radiation on tissues are manifested where this is desirable (i.e. in the tumour itself), but inexorably also in its surrounding area, variously according to the type of radiation, size of the dose, and radiosensitivity of the specific tissue. Side effects appear within a broad timescale, from deterministic effects within the space of a few hours to stochastic effects which may be manifested dozens of years later. In terms of the incidence of side effects on the surrounding structures, radiotherapy of uveal melanoma is particularly worthy of attention, especially due to the risk of damage to structures important for sight, but often also due to the risk of triggering undesirable changes, the consequence of which is loss of the eye as such.
In radiotherapy of uveal melanoma it is necessary to understand and manage also collateral damage, which this as yet irreplaceable therapeutic method inevitably causes. An important component of the strategy of treatment of complications is the prevention of irreversible changes – e.g. in the case of brachytherapy in the form of the use of a radiator adjusted to requirement or more frequently in the excentric placement of the radiator [70].
Retina
Post-radiation changes of the retina are most commonly manifested in the form of vascular incompetence, histologically practically identically as in the case of the vascular system of the tumour [71]. The formation of an irregular capillary lumen takes place, as well as microaneurysms, collaterals develop (Fig. 3). Infiltration leads to retinal edema, and lipid deposits appear. In the further development there is a collapse of the capillary lumen and fibrosis of the capillaries. Exudative retinal detachment as a consequence of massive vascular infiltration occurs upon high doses of radiation, and mostly spontaneously regresses over the course of weeks. Persistent exudative retinal detachment leads to irreversible atrophy of the photoreceptors, which are otherwise radioresistant to a substantial degree as non-replicating structures. Chronic retinal detachment as a consequence of late post-radiation vasculopathy may lead to the development of rubeosis and neovascular glaucoma within the framework of “toxic tumour syndrome”.
Radiation retinopathy is the most common cause of irreversible loss of sight following radiotherapy. It may appear in the form of maculopathy, most often as a consequence of infiltration from typical vascular post-radiation changes of the retina or as a response to ischemic insult upon a background of global vascular incompetence [72]. In therapy the most frequently used methods are laser photocoagulation, photodynamic therapy, transpupillary thermotherapy, intravitreal application of corticosteroids or anti-VEGF antibodies. A series of retrospective studies have been published with the use of anti-VEGF treatment, mostly commenced at the moment of radiotherapy. The majority of studies demonstrate an improvement of radiation induced maculopathy and a reduction of neovascularisation, although this is not always accompanied by an improvement of visual acuity [73].
Retinal pigment epithelium and choroid
The most common initial change in an irradiated RPE is atrophy, loss of melanin, accumulation of lipofuscin and subsequently hyperplasia, which is all partially as a direct consequence of irradiation and partially as a consequence of vascular changes in the adjacent choroid. A practically uniform post-radiation response of the vasculature occurs here – irregular lumen, tortuosity, formation of microaneurysms, infiltration, loss of lumen, neovascularisation, fibrosis. Clinically this most often concerns a zone of atrophy, passing into grainy hypo and hyperpigmentation around the irradiated tissue [74].
Optic nerve
Radiation neuropathy is a frequent cause of severe damage to sight, which in most cases concerns an irreversible condition. Nonetheless, in up to 30 % of cases it is possible to observe a partial spontaneous improvement [75]. The development of direct optic neuropathy usually occurs following absorption of a dose exceeding 50 Gy, the development of pathological changes is contributed to also by vascular changes in the structures of the optic nerve. Therapy of neuropathy of the optic nerve is not yet established or standardised. Hyperbaric oxygen therapy has been used with a limited effect [76]. Systemic corticosteroids are not effective [77], although following their local (i.e. intravitreal) administration an effect has been recorded in the form of a rapid regression of optic nerve edema and a slight improvement of visual functions [78]. Anti-VEGF therapy appears to be promising [79].
Iris
Post-radiation changes of the iris are direct (atrophy and depigmentation) or induced, such as neovascularisation upon a background of global ischemia of the posterior segment, leading to the development of neovascular glaucoma [80], often as a component of toxic tumour syndrome.
Lens
Damage to the lens, i.e. development of cataract following irradiation appears in the case of doses exceeding 8–10 Gy. The lens lamellas are deformed, debris accumulating in the subcapsular region primarily before the posterior capsule worsens transparency [81]. The triggering mechanism is radiation-induced damage to the DNA of the proliferating cells of the lens. Cataract as a consequence of post-radiation changes is a complication which can be resolved by the use of a standard surgical technique. However, at the same time further changes are also generally present, which may complicate the procedure – rubeosis of the iris, secondary glaucoma and posterior synechiae. Perioperative rupture of the posterior capsule is described significantly more frequently [82].
Sclera
Scleromalacia as a consequence of post-radiation changes is a rare complication of treatment of uveal melanoma [83]. The presumed mechanism is again vascular changes – ischemia together with necrotic changes of the adjacent tumour lead to a breach of the scleral structure. A risk factor is therapy of an extensive tumour or damage to the ciliary body [84].
Toxic tumour syndrome
The term toxic tumour syndrome is reserved for a clinical unit composed of exudative retinal detachment, rubeosis of the iris and neovascular glaucoma due to the presence of ischemia and necrotic tumour matter following radiotherapy (Fig. 4). Although this condition may be influenced by all the above therapeutic procedures (laser photocoagulation, photodynamic therapy, transpupillary thermotherapy, intravitreal application of corticosteroids or anti-VEGF preparations), surgical endo- or exoresection of the tumour appears to be the most effective method [85].
Surgical treatment
Surgical treatment of uveal melanoma incorporates a whole range of invasive procedures – from eyeball-preserving procedures on the iris, ciliary body and/or choroid to radical procedures in the form of enucleation of the eyeball and exenteration of the orbit.
Resection of tumour
In the case of melanoma localised purely in the iris, iridectomy is performed, or if applicable iridocyclectomy or iridotrabeculectomy. Anterior-located choroidal melanomas and/or melanomas of the ciliary body (often with affliction of the iris) may be resolved by transscleral resection or exoresection with the aid of partial lamellar transscleral sclerouvectomy. In the case of choroidal melanomas with posterior location, the technique of endoresection with the aid of pars plana vitrectomy (PPV) is used, usually in combination with prior irradiation of the tumour.
Lesions with a height of more than 8 mm [85] are usually indicated for the performance of both exoresection and endoresection of uveal melanoma. In the case of tumours of this size, a poor prognosis persists even after effective radiotherapy with regard to the long-term preservation of the eyeball or visual functions of the eye, and there is a high risk of the development of complications generated by irradiation of the tumour (primarily toxic tumour syndrome). Resection of the tumour furthermore enables the team to obtain material for a histopathological and cytogenetic examination [86] (Fig. 5).
Surgical resection of an intraocular tumour is a technically demanding procedure with potential perioperative and postoperative complications, and should be performed by an experienced retinal surgeon [85].
Iridectomy and iridocyclectomy
Iridectomy is used in the case of lesions which affect only the iris. Sector or peripheral iridectomy is selected according to the location of the lesion. Following conjunctival peritomy, a sclerocorneal flap is created (¾ of the thickness of the sclera/cornea) – a scleral incision is made perilimbally 2–3 mm beyond the limbus, and radial incisions are made 2 mm beyond the border of the tumour. Keratectomy is performed above the area of the tumour in the region of the bed of the sclerocorneal flap. The actual iridectomy is made at a minimum of 1 mm beyond the border of the melanoma, subsequently reconstruction of the pupil is performed, or as the case may be suture of the coloboma of the iris, and the flap and conjunctiva are re-sutured [87].
In the case of melanoma of the iris affecting the root of the iris, anterior partial iridotrabeculocyclectomy is performed. After the creation of a sclerocorneal flap, the anterior chamber is opened by means of a corneal perilimbal incision made in the bed of the flap above the anterior edge of the iris lesion, while radial incisions are made beyond the border of the iridocorneal angle via the sclera into the anterior part of the ciliary body. An incision of the iris is then commenced anteriorly at min. 1 mm beyond the border of the tumour, leading radially to the ciliary sulk. A scleral incision is subsequently made, leading perilimbally in the bed of the sclerocorneal flap, and the block of exscinded tissue can be extracted [87].
In the case of a tumour of the iris affecting the ciliary body, it is suitable to perform complete anterior iridotrabeculcyclectomy. The procedure is again commenced by the creation of a sclerocorneal flap, after which a deep scleral T-incision is made with one incision parallel to the limbus and a second radial incision above the presumed centre of the tumour. The incision is made approx. in ¾ of the thickness of the sclera, and 2 scleral flaps are created along the sides of the radial incision. As in the case of partial iridotrabeculcyclectomy, the anterior chamber is opened and an incision of the iris is made, with the suturing of the iris part of the flap. The procedure continues with the performance of radial incisions leading through the bed of the scleral flaps and pars plicata at a minimum of 1 mm exterior to the sides of the tumour, and with an incision parallel to the limbus leading via the scleral bed and pars plana, beyond the posterior border of the tumour. There follows a suture of the radial scleral part of the sclerocorneal flap and the radial part of the scleral flaps. A perilimbal incision thus remains open between the sclerocorneal flap and the scleral flaps, through which the exscinded tissue together with the tumour is slowly extracted [88,89].
The most common postoperative complications are hypotonia, cataract, hyphaemia, cyclodialysis, dehiscence of the wound and bullous keratopathy [88].
Exoresection
Iridociliary tumours affecting less than 3 hours of the pars plicata and choroidal melanomas with a base smaller than 15 mm are suitable for surgical exoresection [86] (Fig. 6). In addition to post-radiation exoresection of large anterior-located tumours of the choroidea and tumours of the ciliary body, exoresection is indicated in the case of unclear iridociliary lesions with suspicious signs such as growth in size or thickness of the tumour, or pronounced vascularisation. In the case of these lesions it is sometimes not possible to perform a thin-needle biopsy (e.g. in the case of localisation of the lesion in the iridocorneal angle), furthermore different parts of the lesion may manifest different histopathological and cytogenetic characteristics, and the results of an examination of a small sample of tissue may be misleading [89,90].
The procedure is performed in a slight reverse Trendelenburg position, under general anaesthesia with controlled systemic hypotension and monitoring of brain functions. It is commenced by 270° perilimbal peritomy, exposure of the sclera and underpulling of the 3 extraocular rectus muscles around the tumour. If the tumour is localised directly beneath one of the extraocular rectus muscles, the muscle is fixed to a suture and severed. The border of the tumour is subsequently localised and demarcated to the sclera – localisation is performed by translumination of indirect ophthalmoscope with the use of a localiser. The edge of the planned scleral flap is then demarcated on the sclera, overhanging 3–4 mm beyond the border of the tumour, and an incision is made with a sharp knife to a depth of 80–90% of the thickness of the sclera. A scleral lamella is created, which is folded over the limbus. If blue colour does not show through the adjacent choroid, the bed of the scleral lamella is delicately deepened. A perimeter incision is then made through the inner layer of the sclera, approx. 3 mm beyond the border of the tumour, and the area of the uvea is exposed. The uvea is cauterised by bipolar cautery until it turns pale.
In the case of uveal tumours affecting the iris, paracentesis is performed approx. 90° from the main incision, and viscoelastic material is applied into the anterior chamber. The anterior chamber is subsequently opened by a microsurgical knife in the middle of the limbal edge of the scleral flap, and the sclerocorneal incision is extended to the sides.
In the case of melanoma of the ciliary body and/or choroidea, it is usually appropriate to perform limited PPV without infusion in the area of the base of the vitreous body. This reduces the risk of prolapse of the uvea, retina or vitreous body during resection of the tumour. The uveal tumour itself is eventually exscinded with the aid of blunt tip Vannas scissors. First of all the choroidal part or the region of the ciliary body is exscinded, in the case of affliction of the iris, the iris part of the tumour is eventually exscinded. The uveal tumour, together with the adjacent thin scleral lamella, is carefully removed (using a “no-touch” technique) in order to prevent the dissemination of tumour cells into the area of the surgical field. A scleral flap is then sutured into the original position, and the wound is covered by the conjunctiva [86,91,92].
In cases with dominant affliction of the ciliary body, ciliochoroidal and iridociliochoroidal tumours, brachytherapy is performed one month after exoresection (Ru-106 applicator, dose of 100 Gy to tissues 1 mm from the internal surface of the sclera). This reduces the risk of local recurrence of the tumour [86].
The main perioperative complication is an insufficiently strong scleral flap – upon deepening of the scleral bed there is then a danger of perforation of the sclera in the area of the tumour, with the risk of extrascleral dissemination of the tumour. The most common postoperative complications include haemophthalmos, hyphaema, retinal detachment, infiltration in the area of the sceral flap, ablation of the choroidea, subretinal fibrosis, cataract, cystoid macular edema, bullous keratopathy, local recurrence of the tumour or secondary glaucoma [88].
Endoresection
In the case of endoresection, the procedure is not limited by the size of the tumour, but in the case of tumours with a base of more than 15 mm the risk of perioperative and postoperative complications increases (Fig. 7). However, due to poor visualisation, endoresection should be considered in the case of anterior-located tumours, in posterior-located melanomas of the choroid the distance from the optic nerve papilla and fovea should be at least 1 PD [86].
In the case of phakic patients, the procedure is commenced by phacoemulsification with the implantation of an artificial intraocular lens, after which conventional three or four-port PPV is performed, and the posterior hyaloid membrane is thoroughly detached in the area of the tumour. Endodiathermy of the retinal blood vessels is subsequently performed in the area of the base of the tumour. In the case of presence of subretinal fluid, drainage is performed by peripheral retinotomy under perfluorodecalin (PFCI). Retinotomy is performed in the place of the highest prominence of the tumour, via which a vitrectome is inserted into the tumour. There then follows the actual transretinal endoresection of the tumour by the vitrectome. In order to minimise haemorrhage from the blood vessels of the tumour, intraocular pressure is increased in this phase (up to 80 mmHg), and ideally controlled systemic hypotension is induced (systolic pressure under 90 mmHg). Endoresection is performed up to the sclera, the individual scleral and choroidal blood vessels are eventually treated by endolaser or endodiathermy. PCFI is continually supplemented, following endoresection the edges of the retinectomy and choroidectomy are treated with endolaser or by cryocoagulation. A lining of the flat residues of the tumour remains on the bare sclera at the edge of the base of the original tumour. PCFI is subsequently replaced by silicone oil [86,93,94].
Perioperative use of gas tamponade should be carefully considered due to the risk of air embolism into the brain, which may have fatal consequences [95,96]. A common perioperative and postoperative complication is haemorrhage, perioperatively it is possible to control this by means of increasing intraocular pressure or by targeted endodiathermy [86]. A potential risk of endoresection is perioperative spread of vital tumour cells. For this reason, the standard recommendation is for a combined approach with irradiation of the tumour before endoresection [97]. The risk of tumour dissemination during endoresection is disputed e.g. by Damato, who due to the risk of post-radiation complications (retinopathy, neuropathy, maculopathy and above all toxic tumour syndrome) performs endoresection also without prior radiotherapy [98].
Enucleation
Enucleation is the method of choice in the case of advanced uveal melanomas which cannot be treated effectively by teleradiotherapy and/or cause complete or practical loss of visual acuity, total retinal detachment of painful secondary glaucoma [99].
At the beginning of the procedure a 360° peritomy of the conjunctiva is performed, with exposure of the sclera. Following fixation the extraocular rectus muscles are separated from the wall of the eyeball, and the eyeball is sutured with a firm fixing scleral suture. After the severing of the optic nerve beyond the eyeball with enucleation scissors (from an inferonasal approach) and halting of bleeding through a combination of compression and haemostatic implant, a biocompatible porous implant made of hydroxyapatite or permeable highly dense polyethylene is inserted into the enucleation cavity. In case of necessity it is possible to adjust the shape of the polyethylene implant individually with a scalpel following placing in a hot sterile saline solution. The extraocular muscles are fixed above the implant, which can also be coated in a vicryl mesh or inserted into a bed from the donor’s sclera, and the extraocular rectus muscles can then be fixed directly to this coating. The enucleation cavity is closed in 2 layers via the Tenon’s membrane and the conjunctiva. Approximately 4 – 6 weeks after enucleation it is possible to begin using an acrylate or glass eye replacement produced to order.
Exenteration
Exenteration of the orbit is indicated for patients with an advanced tumour with extrabulbar dissemination or in the case of recurrence of a tumour in the orbit following prior enucleation. If the local finding so allows, a technique preserving the eyelids is used (Fig. 8).
At the beginning of the procedure the upper and lower eyelids are fixed by a suture passing through the skin, the orbicularis oculi muscle and the tarsal plate. A skin incision is then made parallel to the edge of the eyelid, 2 mm above and beneath the line of the eyelashes. The incision is made through the skin and the orbicularis oculi muscle, which are bluntly separated upward and downward, exposing the periosteum circularly beyond the edge of the orbit. An incision is then made approx. 2 mm exterior to the edge of the orbit, and the bone is exposed. The periosteum is carefully separated from the bone wall of the orbit using a raspatorium. After separation of the periosteum up to the apex of the orbit, the tissue is exscinded as close as possible to the orbital apex with the aid of enucleation scissions (approach from inferonasal side) and extracted using a fixating eyelid suture performed at the beginning of the procedure. There follows intensive compression, after which the exenteration cavity is reviewed and any residues of soft tissues are removed. Following complete haemostasis a sleeve drain is inserted into the orbit and the skin of the eyelids is sutured. Approximately 6–8 weeks after the procedure it is possible to fit an orbital prosthesis [100].
New prospects in the therapy of uveal melanoma
Although radiotherapy of uveal melanoma, if applicable in combination with surgical treatment, ensures good control over the tumour and enables the preservation of the eyeball, it is often linked with a significant decrease of visual acuity. There is therefore an endeavour to find an effective conservative therapeutic approach which could provide better options for saving the visual functions of the affected eye.
In the case of small choroidal melanomas, a method using light-activated molecules of the pharmaceutical AU-011 appears to be promising. AU-011 is composed of synthetic, virus-like particles, derived from human papillomaviruses (HPV). The medicament is applied to the eye by intravitreal injection, and in the eye selectively binds to cells of the uveal melanoma and other tumour cells, which excessively exprimate heparan sulphate proteoglycans. After activation by a diode laser with a wavelength of 689 nm, small AU-011 molecules selectively destroy the cellular membrane of the tumour cells [101].
Zdroje
1. Damato B, Heimann H. Personalized treatment of uveal melanoma. Eye. 2013 Feb;27(2):172–179.
2. Shields CL, Furuta M, Thangappan A et al. Metastasis of uveal melanoma millimeter-by-millimeter in 8033 consecutive eyes. Arch Ophthalmol. 2009 Aug;127(8):989–998.
3. Jouhi S, Jager MJ, de Geus SJR et al. The Small Fatal Choroidal Melanoma Study. A Survey by the European Ophthalmic Oncology Group. Am J Ophthalmol. 2019 Jun;202:100-108.
4. Bechrakis NE, Sehu KW, Lee WR, Damato BE, Foerster MH. Transformation of cell type in uveal melanomas: a quantitative histologic analysis. Arch Ophthalmol. 2000 Oct;118(10):1406–1412.
5. Frizziero L, Midena E, Trainiti S et al. Uveal Melanoma Biopsy: A Review. Cancers. 2019 Aug;11(8):1075. doi:10.3390/cancers11081075
6. Bagger MM. Intraocular biopsy of uveal melanoma Risk assessment and identification of genetic prognostic markers. Acta Ophthalmol. 2018 Jul;96:1–28.
7. Qiang Z, Cairns JD. Laser photocoagulation treatment of choroidal melanoma. Aust N Z J. Ophthalmol. 1993 May;21(2):87–92.
8. Mashayekhi A, Shields CL, Rishi P et al. Primary transpupillary thermotherapy for choroidal melanoma in 391 cases: importance of risk factors in tumor control. Ophthalmology 2015 Mar;122(3):600–609.
9. Shields CL, Shields JA, Perez N, Singh AD, Cater J. Primary transpupillary thermotherapy for small choroidal melanoma in 256 consecutive cases: outcomes and limitations. Ophthalmology. 2002 Feb;109(2):225–234.
10. Aaberg TM Jr, Bergstrom CS, Hickner ZJ, Lynn MJ. Long-term results of primary transpupillary thermal therapy for the treatment of choroidal malignant melanoma. Br J Ophthalmol 2008 Jun;92(6):741–746.
11. Shields CL, Cater J, Shields JA et al. Combined plaque radiotherapy and transpupillary thermotherapy for choroidal melanoma in 270 consecutive patients. Arch Ophthalmol 2002 Jul;120(7):933–940.
12. Cerman E, Çekiç O. Clinical use of photodynamic therapy in ocular tumors. Surv Ophthalmol 2015 Nov-Dec;60(6):557–574.
13. Avery RB, Diener-West M, Reynolds SM, Grossniklaus HE, Green WR, Albert DM. Histopathologic characteristics of choroidal melanoma in eyes enucleated after iodine 125 brachytherapy in the collaborative ocular melanoma study. Arch Ophthalmol. 2008 Feb;126(2):207–212.
14. Brewington BY, Shao YF, Davidorf FH, Cebulla CM. Brachytherapy for patients with uveal melanoma: historical perspectives and future treatment directions. Clin Ophthalmol. 2018 May;12:925-934.
15. Deutschmann R. Ueber intraokularen Tumor und Strahlentherapie. Augenheilkd. 1915;33:206–209. German.
16. Moore RF. Choroidal sarcoma treated by intraocular insertion of radon seeds. Br J Ophthalmol. 1930 Apr;14(4):145–152.
17. Stallard HB. A new technique for the application of radon seeds to the sclera in the treatment of glioma retinae. Br J Ophthalmol. 1938 Oct;22(10):604–608.
18. Shields CL, Shields JA, Cater J et al. Plaque Radiotherapy for Uveal Melanoma: Long-term Visual Outcome in 1106 Consecutive Patients. Arch Ophthalmol. 2000 Sep;118(9):1219–1228.
19. Lommatzsch P. Morphologische und funktionelle Veränderungen des Kaninchenauges nach Einwirkung von Betastrahlen (106Ru/106Rh) auf den dorsalen Bulbusabschnitt. [Morphological and functional changes in the rabbit eye on exposure of the dorsal bulb section to beta rays (106Ru/106Rh)]. Von Graefes Arch Klin Exp Ophthalmol. 1968;176:100–125. German.
20. Damato B, Patel I, Campbell IR, Mayles HM, Errington RD. Local tumor control after 106Ru brachytherapy of choroidal melanoma. Int J Radiat Oncol Biol Phys. 2005 Oct;63(2):385–291.
21. Bergman L, Nilsson B, Lundell G, Lundell M, Seregard S. Ruthenium brachytherapy for uveal melanoma, 1979-2003: survival and functional outcomes in the Swedish population. Ophthalmology. 2005 May;112(5):834–840.
22. Barker CA, Francis JH, Cohen GN et al. 106Ru plaque brachytherapy for uveal melanoma: factors associated with local tumor recurrence. Brachytherapy. 2014 Nov-Dec;13(6):584–590.
23. Tarmann L, Wackernagel W, Avian A et al. Ruthenium-106 plaque brachytherapy for uveal melanoma. Br J Ophthalmol. 2015 Dec;99(12):1644–1649.
24. Earle J, Kline RW, Robertson DM. Selection of iodine 125 for the Collaborative Ocular Melanoma Study. Arch Ophthalmol. 1987 Jun;105(6):763–764.
25. Packer S, Rotman M, Fairchild RG, Albert DM, Atkins HL, Chan B. Irradiation of choroidal melanoma with iodine 125 ophthalmic plaque. Arch Ophthalmol. 1980 Aug;98(8):1453–1457.
26. Newman GH, Davidorf FH, Havener WH, Makley TA. Conservative management of malignant melanoma – I: irradiation as a method of treatment for malignant melanoma of the choroid. Arch Ophthalmol. 1970 Jan;83(1):21–26.
27. Stallard HB. Radiotherapy for malignant melanoma of the choroid. Br J Ophthalmol. 1966 Mar;50(3):147–155.
28. Shields JA, Augsburger JJ, Brady LW, Day JL. Cobalt plaque therapy of posterior uveal melanomas. Ophthalmology 1982 Oct;89(10):1201–1207.
29. Cruess AF, Augsburger JJ, Shields JA, Brady LW, Markoe AM, Day JL. Regression of posterior uveal melanomas following cobalt-60 plaque radiotherapy. Ophthalmology. 1984 Dec;91(12):1716–1719.
30. Finger PT, Chin KJ, Duvall G. Palladium-103 ophthalmic plaque radiation therapy for choroidal melanoma: 400 treated patients. Ophthalmology. 2009 Apr;116(4):790–796.
31. van Ginderdeuren R, van Limbergen E, Spileers W. 18 years experience with high dose rate strontium-90 brachytherapy of small to medium sized posterior uveal melanoma. Br J Ophthalmol. 2005 Oct;89(10):1306–1310.
32. Henderson MA, Shirazi H, Lo SS et al. Stereotactic Radiosurgery and Fractionated Stereotactic Radiotherapy in the Treatment of Uveal Melanoma. Technol Cancer Res Treat. 2006 Aug;5(4):411–419.
33. Zehetmayer M, Kitz K, Menapace R et al. Local Tumor Control and Morbidity After One to Three Fractions of Stereotactic External Beam Irradiation for Uveal Melanoma. Radiother. Oncol. 2000 May;55(2):135–144.
34. Marchini G, Gerosa M, Piovan E et al. Gamma Knife Stereotactic Radiosurgery for Uveal Melanoma: Clinical Results after 2 Years. Stereotact Funct Neurosurg.1996;66 Suppl 1:208–213.
35. Langmann G, Pendl G, Klaus M, Papaefthymiou G, Guss H. Gamma Knife Radiosurgery for Uveal Melanomas: An 8-year Experience. J Neurosurg. 2000 Dec;93 (Suppl 3):184–188.
36. Langmann G, Pendl G, Mullner K, Feichtinger KH, Papaefthymiouaf G. High-compared with Low-dose Radiosurgery for Uveal Melanomas. J Neurosurg. 2002 Dec;97(Suppl 5):640–643.
37. Backlund EO. The history and development in radiosurgery. In: Stereotactic Radiosurgery Update. Proceedings of the International Stereotactic Radiosurgery Symposium, Pittsburg (USA): Elsevier Science NewYork;1991, p.1–7.
38. Liščák R. a kolektiv. Radiochirurgie gama nožem-Principy a neurochirurgické aplikace, 1st ed. Praha (Czech republic): Grada Publishing;2011. Chapter 2, Novotný J. Fyzikální principy radiochirurgie; p.19–31. Czech.
39. Langmann G, Pendl G, Schrottner O. Gamma knife of uveal melanoma radiosurgery for intraocular melanomas. Preliminary report. Spectrum Augenhelkd 1995;9 (Suppl 1):16–21.
40. Bellmann C, Fuss M, Holz F et al. Stereotactic radiation therapy for malignant choroidal tumors, preliminary, short-term results. Ophtalmology 2000 Feb;107(2):358–365.
41. Simonova G, Novotny J, Liscak R, Pilbauer J. Leksell Gamma Knife Treatment of Uveal Melanoma. J Neurosurg. 2002 Dec;97(Suppl 5):635–639.
42. Sarici AM, Pazarli H. Gamma-knife-based stereotactic radiosurgery for medium-and large-sized posterior uveal melanoma. Graefes Arch Clin Exp Ophthalmol. 2013 Jan;251(1):285–294.
43. Modorati G, Miserocchi E, Galli L, Picozzi P, Rama P. Gamma knife radiosurgery for uveal melanoma: 12 years of experience. Br J Ophthalmol. 2009 Jan;93(1):40–44.
44. Joye RP, Williams LB, Chan MD et al. Local control and results of Leksell Gamma Knife therapy for the treatment of uveal melanoma. Ophthalmic Surg Lasers Imaging Retina. 2014 Mar-Apr;45(2):125–131.
45. Kang DW, Lee SC, Park YG, Chang JH. Longterm results of GammaKnife surgery for uveal melanomas. J Neurosurg. 2012 Dec;117 Suppl:108–14.
46. Cyberknife. Mojemedicina.cz [Internet]. Roche Czech Republic;2020 [cited 2020 Jan 23]. Available from: https://www.mojemedicina.cz/pruvodce-pacienta/lecebne-metody/cyberknife-1.html. Czech.
47. Zkušenosti a výsledky extrakraniální stereotaktické radioterapie přístrojem CyberKnife - Zdraví.Euro.cz. Zdravotnictví a medicína - Zdraví.Euro.cz [Internet]. Mladá Fronta a.s.;2020 [cited 2020 Jan 23]. Available from: https://zdravi.euro.cz/clanek/postgradualni-medicina/zkusenosti-a-vysledky-extrakranialni-stereotakticke-radioterapie-pristrojem-cyberknife-481954. Czech.
48. Brain Tumor Treatment Process Accuray CyberKnife. Home Accuray CyberKnife [Internet]. Accuray;2020 [cited 2020 Jan 23]. Available from: https://www.cyberknife.com/treatment/process/brain%20tumor.
49. Výhody a omezení léčby, indikace - Kybernetický nůž Cyberknife. Kybernetický nůž Cyberknife [Internet]. Fakultní nemocnice Ostrava;2009 [cited 2020 Jan 23]. Available from: https://cyberknife.fno.cz/cs/clanky/vyhody-a-omezeni-lecby-indikace. Czech.
50. Eibl-Lindner K, Furweger C, Nentwich M et al. Robotic radiosurgery for the treatment of medium and large uveal melanoma. Melanoma Res 2016 Feb; 6(1):51-57.
51. Soykut ED, Guney YY, Dizman A et al. Retrospective review of uveal melanoma patients treated with fractionated stereotactic radiotherapy. Cumhuriyet Medical Journal 2018 Dec;40(4):421–431.
52. Klop LA, Faase –De Hoog M, Luthart L, de Pan C. Stereotactic radiosurgery of uveal melanoma on the Cyberknife M6TM [Internet]. Available from: https://www.macromedics.com/files/upload/07/dsps-eye-melanoma-poster-emc-nvmbr-congress.pdf.
53. Zorlu F, Selek U, Kiratli H. Initial results of fractionated CyberKnife radiosurgery for uveal melanoma. J Neurooncol 2009 Aug; 94(1):111–117.
54. Fry DW, Harvie RD, Mullett LB, Walkinshaw W. A traveling wave linear accelerator for 4 MeV electrons. Nature 1948 Nov;162(4126):859–861.
55. Betti OO, Derechinski VE. Hyperselective encephalic irradiation with a linear accelerator. Acta Neurochirurgica Supplement 1984; 33:385–390.
56. Winston KR, Lutz W. Linear accelerator as a neurosurgical tool for stereotactic radiosurgery. Neurosurgery 1988 Mar; 22(3):454–464.
57. Suesskind D, Scheiderbauer J, Buchgeister M et al. Retrospective evaluation of patients with uveal melanoma treated by stereotactic radiosurgery with and without tumor resection. Jama Ophthalmol. 2013 May;131(5):630–637.
58. Furdova A, Sramka M., Chorvath M, Kralik G. Linear accelarator stereotactic radiosurgery in intraocular malignant melanoma. Austin J Radiat Oncol Cancer 2015;1(2):1008.
59. Thornton AF, Fitzek M, Klein S et al. Proton beam radiotherapy: a specialized treatment alternative. Community Oncology. 2007 Oct;4(10):599–607.
60. Levin WP, Kooy H, Loeffler JS, DeLaney TF. Proton therapy. Br J Cancer. 2005 Oct 17;93(8):849-854.
61. Fleurette F, Charvet-Protat S. Proton- et neutronthérapie dans le traitement du cancer: considérations cliniques et économiques. [Proton and neutron radiation in cancer treatment: clinical and economic outcomes]. Bull Cancer Radiother 1996;83(1),223-27. French.
62. Damato B, Kacperek A, Chopra M, Campbell IR, Errington RD. Proton beam radiotherapy of choroidal melanoma: the Liverpool-Clatterbridge experience. Int J Radiat Oncol Biol Phys. 2005 Aug;62(5):1405–1411.
63. Courdi A, Caujolle JP, Grange JD et al. Results of proton therapy of uveal melanomas treated in Nice. Int J Radiat Oncol Biol Phys. 1999 Aug 1;45(1):5-11.
64. Kishan AU, Modjtahedi BS, Morse LS, Lee P: Radiation Therapy for Neovascular Age-related Macular Degeneration. Int J Radiat Oncol Biol Phys. 2013 Mar;85(3):583–597.
65. Fuss M, Loredo LN, Blacharski PA, Grove RI, Slater JD. Proton radiation therapy for medium and large choroidal melanoma: preservation of the eye and its functionality. Int J Radiat Oncol Biol Phys. 2001 Mar 15;49(4):1053–1059.
66. Amstutz CA, Bechrakis NE, Foerster MH, Heufelder J, Kowal JH. Intraoperative localization of tantalum markers for proton beam radiation of choroidal melanoma by an opto-electronic navigation system: a novel technique. Int J Radiat Oncol Biol Phys. 2012 Mar;82(4):1361–1366.
67. Marnitz S, Cordini D, Bendl R et al. Proton therapy of uveal melanomas: intercomparison of MRI-based and conventional treatment planning. Strahlenther Onkol. 2006 Jul;182(7):395–399.
68. Damato B, Kacperek A, Chopra M, Sheen MA, Campbell IR, Errington RD. Proton beam radiotherapy of iris melanoma. Int J Radiat Oncol Biol Phys. 2005 Sep;63(1):109–115.
69. Caujolle JP, Mammar H, Chamorey E, Pinon F, Herault J, Gastaud P. Proton beam radiotherapy for uveal melanomas at nice teaching hospital: 16 years' experience. Int J Radiat Oncol Biol Phys. 2010 Sep;78(1):98–103.
70. Russo A, Laguardia M, Damato B. Eccentric ruthenium plaque radiotherapy of posterior choroidal melanoma. Graefes Arch Clin Exp Ophthalmol. 2012 Oct;250(10):1533–1540.
71. Archer DB, Gardiner TA. Ionizing radiation and the retina. Curr Opin Ophthalmol. 1994 Jun;5(3):59–65.
72. Zahorjanova P, Furdova A, Waczulikova I, Sramka M, Kralik G, Stubna M. Radiation maculopathy after one – day session stereotactic radiosurgery in patients with ciliary body and choroidal melanoma. Čes a slov. Oftal. 2019;75(1):3–10.
73. Giuliari GP, Sadaka A, Hinkle DM, Simpson ER. Current treatments for radiation retinopathy. Acta Oncol. 2011 Jan;50(1):6–13.
74. Amoaku WM, Lafaut B, Sallet G, De Laey JJ. Radiation choroidal vasculopathy: an indocyanine green angiography study. Eye 1995;9(Pt 6):738–744.
75. Kim IK, Lane AM, Egan KM, Munzenrider J, Gragoudas ES. Natural history of radiation papillopathy after proton beam irradiation of parapapillary melanoma. Ophthalmology. 2010;117(8):1617–1622.
76. Borruat FX, Schatz NJ, Glaser JS, Feun LG, Matos L. Visual recovery from radiation-induced optic neuropathy. The role of hyperbaric oxygen therapy. J Clin Neuroophthalmol. 1993 Jun;13(2):98–101.
77. Girkin CA, Comey CH, Lunsford LD, Goodman ML, Kline LB. Radiation optic neuropathy after stereotactic radiosurgery. Ophthalmology. 1997;104(10):1634–1643.
78. Shields CL, Demirci H, Marr BP et al. Intravitreal triamcinolone acetonide for acute radiation papillopathy. Retina. 2006 May-Jun;26(5):537–544.
79. Finger PT, Chin KJ. Antivascular endothelial growth factor bevacizumab for radiation optic neuropathy: secondary to plaque radiotherapy. Int J Radiat Oncol Biol Phys. 2012 Feb;82(2):789–798.
80. Cappin JM. Malignant melanoma and rubeosis iridis. Histopathological and statistical study. Br J Ophthalmol. 1973 Nov;57(11):815–824.
81. Merriam GR, Worgul BV. Experimental radiation cataract-- its clinical relevance. Bull N Y Acad Med. 1983 May;59(4):372–392.
82. Wachtlin J, Bechrakis NE, Schueler AO, Helbig H, Bornfeld N, Foerster MH. Phacoemulsification following treatment of choroidal melanoma. Graefes Arch Clin Exp Ophthalmol. 2000 Dec;238(12):942–948.
83. Radin PP, Lumbroso-Le Rouic L, Levy-Gabriel C, Dendale R, Sastre X, Desjardins L. Scleral necrosis after radiation therapy for uveal melanomas: report of 23 cases. Graefes Arch Clin Exp Ophthalmol. 2008 Dec;246(12):1731–1736.
84. Gunduz K, Shields CL, Shields JA, Cater J, Freire JE, Brady LW. Plaque radiotherapy of uveal melanoma with predominant ciliary body involvement. Arch Ophthalmol. 1999 Feb;117(2):170–177.
85. Hamza HS, Elhusseiny AM. Choroidal Melanoma Resection. Middle East Afr J Ophthalmol. 2018 Apr-Jun;25(2):65–70.
86. Gündüz K, Bechrakis NE. Exoresection and endoresection for uveal melanoma. Middle East Afr J Ophthalmol. 2010 Jul;17(3):210–216.
87. Klauber S, Jensen PK, Prause JU, Kessing SV. Surgical treatment of iris and ciliary body melanoma: follow-up of a 25-year series of patients. Acta Ophthalmol. 2012 Mar;90(2):122–6.
88. Rospond-Kubiak I, Damato B. The surgical approach to the management of anterior uveal melanomas. Eye. 2014 Jun;28(6):741–747.
89. Marigo FA, Finger PT. Anterior segment tumors: Current concepts and innovations. Surv Ophthalmol. 2003 Nov-Dec;48(6):569–593.
90. Damato B, Woulds WS. Indications for trans-scleral local resection of uveal melanoma. Br J Ophthalmol. 1996 Nov;80(11):1029–1030.
91. Shields JA, Shields CL. Surgical approach to lamellar sclerouvectomy for posterior uveal melanomas: The 1986 Schoenberg lecture. Ophthalmic Surg. 1988 Nov;19(11):774–780.
92. Shields JA, Shields CL, Shah P, Sivalingam V. Partial lamellar sclerouvectomy for ciliary body and choroidal tumors. Ophthalmology. 1991 Jun;98(6):971–983.
93. Damato B, Jones AG. Uveal melanoma: resection techniques. Ophthalmol Clin North Am. 2005 Mar;18(1):119–128.
94. Damato B, Groenewald C, McGalliard J, Wong D. Endoresection of choroidal melanoma. Br J Ophthalmol. 1998 Mar;82(3):213–218.
95. Rice JC, Liebenberg L, Scholtz RP, Torr G. Fatal air embolism during endoresection of choroidal melanoma. Retin Cases Brief Rep 2014;8(2):127–129.
96. Joussen AM, Wong D. Egress of large quantities of heavy liquids from exposed choroid: a route for possible tumor dissemination via vortex veins in endoresection of choroidal melanoma. Graefes Arch Clin Exp Ophthalmol. 2015 Feb;253(2): 177–178.
97. Bechrakis NE, Foerster MH. Neoadjuvant proton beam radiotherapy combined with subsequent endoresection of choroidal melanomas. Int Ophthalmol Clin. 2006;46(1):95–107.
98. Damato B. Endoresection of uveal melanoma without radiotherapy. Acta Ophthalmol, 2017;95.
99. Shields JA, Shields CL. Management of posterior uveal melanoma: past, present, and future: the 2014 Charles L. Schepens lecture. Ophthalmology. 2015 Feb;122(2):414–428.
100. Shields JA, Shields CL, Suvarnamani C, Tantasira M, Shah P: Orbital exenteration with eyelid sparing: Indications, technique and results. Ophthalmic Surg. 1991 May;22(5):292–297.
101. Singh AD. Light-Activated Therapy of Choroidal Melanoma. A new modality could be an effective alternative to radiation. Retinal Physician. 2019 Jul;16:41–45.
Štítky
OftalmológiaČlánok vyšiel v časopise
Česká a slovenská oftalmologie
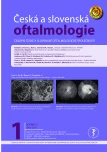
2021 Číslo 1
- Cyklosporin A v léčbě suchého oka − systematický přehled a metaanalýza
- Myasthenia gravis: kombinace chirurgie a farmakoterapie jako nejefektivnější modalita?
- Pomocné látky v roztoku latanoprostu bez konzervačních látek vyvolávají zánětlivou odpověď a cytotoxicitu u imortalizovaných lidských HCE-2 epitelových buněk rohovky
- Konzervační látka polyquaternium-1 zvyšuje cytotoxicitu a zánět spojený s NF-kappaB u epitelových buněk lidské rohovky
- Dlouhodobé výsledky lokální léčby cyklosporinem A u těžkého syndromu suchého oka s 10letou dobou sledování
Najčítanejšie v tomto čísle
-
TERAPIE UVEÁLNÍHO MELANOMU
PŘEHLED - BLOW-IN FRAKTURA STROPU OČNICE KAZUISTIKA
- STAVY NAPODOBUJÍCÍ PACHYCHOROIDNÍ CHOROBY SÍTNICE – SOUBOR KAZUISTIK
- VYŠETŘENÍ ZORNÉHO POLE U HYPERTENZNÍCH GLAUKOMŮ