Low Soluble Syndecan-1 Precedes Preeclampsia
Introduction:
Syndecan-1 (Sdc1; CD138) is a major transmembrane heparan sulfate proteoglycan expressed on the extracellular, luminal surface of epithelial cells and syncytiotrophoblast, thus comprising a major component of the glycocalyx of these cells. The “soluble” (shed) form of Sdc1 has paracrine and autocrine functions and is normally produced in a regulated fashion. We compared plasma soluble Sdc1 concentrations, in relation to placental Sdc1 expression, in uncomplicated (control) and preeclamptic pregnancies.
Methods:
We evaluated soluble Sdc1 across uncomplicated pregnancy, and between preeclamptic, gestational hypertensive and control patients at mid-pregnancy (20 weeks) and 3rd trimester by ELISA. Placental expression level of Sdc1 was compared between groups in relation to pre-delivery plasma soluble Sdc1. Participants were recruited from Magee-Womens Hospital.
Results:
In uncomplicated pregnancy, plasma soluble Sdc1 rose significantly in the 1st trimester, and reached an approximate 50-fold increase at term compared to post pregnancy levels. Soluble Sdc1 was lower at mid-pregnancy in women who later developed preeclampsia (P<0.05), but not gestational hypertension, compared to controls, and remained lower at late pregnancy in preeclampsia (P<0.01) compared to controls. Sdc1 was prominently expressed on syncytiotrophoblast of microvilli. Syncytiotrophoblast Sdc1 immunostaining intensities, and mRNA content in villous homogenates, were lower in preeclampsia vs. controls (P<0.05). Soluble Sdc1 and Sdc1 immunostaining scores were inversely associated with systolic blood pressures, and positively correlated with infant birth weight percentile.
Conclusion:
Soluble Sdc1 is significantly lower before the clinical onset of preeclampsia, with reduced expression of Sdc1 in the delivered placenta, suggesting a role for glycocalyx disturbance in preeclampsia pathophysiology.
Authors:
Robin E. Gandley 1,2; Andrew Althouse 1; Arundhathi Jeyabalan 1,2,3; Julia M. Bregand-White 2; Stacy Mcgonigal 1; Ashley C. Myerski 1; Marcia Gallaher 1; Robert W. Powers 1,2; Carl A. Hubel 2*
Authors place of work:
Magee-Womens Research Institute, University of Pittsburgh, Pittsburgh, Pennsylvania, United States of America
1; Department of Obstetrics, Gynecology & Reproductive Sciences, Division of Maternal Fetal Medicine, University of Pittsburgh, Pittsburgh, Pennsylvania, United States of America
2; Clinical and Translational Research Institute, University of Pittsburgh, Pittsburgh, Pennsylvania, United States of America
3
Published in the journal:
PLoS ONE 11(6)
Category:
Research article
© 2016 Gandley et al. This is an open access article distributed under the terms of the Creative Commons Attribution License, which permits unrestricted use, distribution, and reproduction in any medium, provided the original author and source are credited.
The electronic version of this article is the complete one and can be found online at: http://journals.plos.org/plosone/article?id=10.1371%2Fjournal.pone.0157608
Summary
Introduction:
Syndecan-1 (Sdc1; CD138) is a major transmembrane heparan sulfate proteoglycan expressed on the extracellular, luminal surface of epithelial cells and syncytiotrophoblast, thus comprising a major component of the glycocalyx of these cells. The “soluble” (shed) form of Sdc1 has paracrine and autocrine functions and is normally produced in a regulated fashion. We compared plasma soluble Sdc1 concentrations, in relation to placental Sdc1 expression, in uncomplicated (control) and preeclamptic pregnancies.
Methods:
We evaluated soluble Sdc1 across uncomplicated pregnancy, and between preeclamptic, gestational hypertensive and control patients at mid-pregnancy (20 weeks) and 3rd trimester by ELISA. Placental expression level of Sdc1 was compared between groups in relation to pre-delivery plasma soluble Sdc1. Participants were recruited from Magee-Womens Hospital.
Results:
In uncomplicated pregnancy, plasma soluble Sdc1 rose significantly in the 1st trimester, and reached an approximate 50-fold increase at term compared to post pregnancy levels. Soluble Sdc1 was lower at mid-pregnancy in women who later developed preeclampsia (P<0.05), but not gestational hypertension, compared to controls, and remained lower at late pregnancy in preeclampsia (P<0.01) compared to controls. Sdc1 was prominently expressed on syncytiotrophoblast of microvilli. Syncytiotrophoblast Sdc1 immunostaining intensities, and mRNA content in villous homogenates, were lower in preeclampsia vs. controls (P<0.05). Soluble Sdc1 and Sdc1 immunostaining scores were inversely associated with systolic blood pressures, and positively correlated with infant birth weight percentile.
Conclusion:
Soluble Sdc1 is significantly lower before the clinical onset of preeclampsia, with reduced expression of Sdc1 in the delivered placenta, suggesting a role for glycocalyx disturbance in preeclampsia pathophysiology.
Introduction
The syncytiotrophoblast is a specialized, multinucleate epithelium formed by the fusion of cytotrophoblasts within the placental villi. With its continuous exchange surface interfacing with maternal blood, the syncytiotrophoblast microvillous membrane has critical functions including transport of nutrients to the fetus [1]. Deficient uterine spiral artery remodeling (failure of spiral arteries to transform to high-flow, low-resistance conduits), frequently seen with preeclampsia or fetal growth restriction (FGR), is thought to result in reduced or intermittent blood flow to the intervillous space leading to syncytiotrophoblast dysfunction and damage [2].
The surface of eukaryotic cells has a hydrated matrix of specialized proteoglycans and glycoproteins, linked to the plasma membrane, known as the glycocalyx [3,4] The vascular endothelial glycocalyx is a critical regulator of vascular function, and endothelial glycocalyx damage has been implicated in reperfusion oxidative injury, inflammation, atherosclerosis and diabetes [3,5–10]. The syncytiotrophoblast microvillous membrane surface also has a substantial glycocalyx [11–13] but its functions are poorly understood.
Syndecan-1 (Sdc1; CD138) is a major transmembrane heparan sulfate proteoglycan expressed on the surface (glycocalyx) of epithelial cells, endothelial cells and plasma cells[14,15]. Sdc1 regulates diverse cell behaviors including adhesion, proliferation, motility, intracellular signaling, growth factor and macromolecular cell surface binding and cellular internalization, angiogenesis, lipid metabolism, wound healing, regulation of leukocyte migration, and endothelial responses to fluid shear stress [14,16–20]. Sdc1 is strongly expressed on villus STB microvillous membrane, but not on cytotrophoblast or stroma, extravillous trophoblast, nor fetal vascular endothelium [21–27]. Expression of Sdc1 on the STB microvillous membrane is reportedly reduced in preeclampsia [21,22,25] and iatrogenic IUGR with fetal compromise[26], compared to uncomplicated pregnancy.
Extracellular domains of Sdc1 are constitutively shed from the glycocalyx, typically in processes regulated by sheddases (e.g., matrix metalloproteinases and heparanase)[3,28]. The soluble form of Sdc1 is thought to have important functions as a paracrine and autocrine effector, and competitor of transmembrane Sdc1 for growth factors and other extracellular ligands [14,15,20]. Sdc1 shedding is an important response in wound healing with effects on inflammation, proliferation and remodeling [3,15,20]. Circulating soluble Sdc1 concentrations can be increased by excess reactive oxygen species or inflammatory stimuli, as exemplified by the extremely high values observed with sepsis [3,10,28,29].
Soluble Sdc1 concentrations have not been systematically examined in uncomplicated (normal) or preeclamptic pregnancies. Our initial hypothesis was that plasma soluble Sdc1 levels would increase with advancing normal pregnancy, and increase significantly more so before and after the onset of preeclampsia in association with reduction of Sdc1 immunoreactivity on STB of villous placenta. The rationale was based on the concept that the increased systemic inflammation thought to underlie the pathogenesis of preeclampsia, representing an accentuation of the normal inflammatory response to pregnancy [30], might be a stimulus for increased Sdc1 shedding. We first measured soluble Sdc1 longitudinally in maternal plasma from uncomplicated pregnancies, by trimester and proximally postpartum. We then investigated if concentrations of soluble Sdc1 were different at 20 weeks’ gestation, before clinical onset of disease, and late pregnancy after disease onset, in women who later developed preeclampsia compared to normotensive controls and women who later developed gestational hypertension (without proteinuria). In a different set of preeclampsia cases and controls we compared villous placental Sdc1 expression profile, and looked for correlations with clinical variables and plasma Sdc1. Finally, to test for lasting constitutional differences, we measured plasma soluble Sdc1 one year after delivery, comparing non-pregnant women with a history of preeclampsia to women with a history of uncomplicated pregnancy.
Methods
Comparison groups
We conducted a nested, case-control study of pregnant women enrolled between 1999 and 2012 as part of an ongoing longitudinal and cross-sectional investigation of preeclampsia (Pregnancy Exposures and Preeclampsia Prevention Study; PEPP) at Magee-Womens Hospital and Magee-Womens Research Institute, Pittsburgh, PA, USA. Written informed consent was obtained from all study subjects. The University of Pittsburgh Institutional Review Board approved the study. The study participants had also given permission to be contacted for additional preeclampsia-related research studies upon consenting to participate in the PEPP Project. A subset women enrolled in PEPP were thus evaluated 1 year after pregnancy, with separate University of Pittsburgh IRB approval and written informed consent.
We studied 5 different subsets of patients as follows. These groups were mostly non-overlapping, reflecting comparison criteria.
- We evaluated gestational changes in soluble Sdc1 using longitudinal maternal plasma samples from 8 women with uncomplicated pregnancy, at gestational weeks 7–11, 17–26, 37–41; and 24–48 hours postpartum and 4–9 weeks postpartum. Patient characteristics are given in S1 Table.
- Comparisons of plasma soluble Sdc1 at mid-pregnancy were performed. Nine women in this study set developed preeclampsia later in the pregnancy, 9 developed gestational hypertension (without proteinuria) later in the pregnancy, and 19 had an uncomplicated pregnancy outcome (characteristics summarized in Table 1). The samples were matched for gestational age (mean gestational weeks ~20, range 18–24). Gestational age-matched 3rd trimester samples were not sufficiently available from these patients.
- We compared 17 women with preeclampsia to 17 with uncomplicated pregnancy, and 8 women with gestational hypertension to 8 women with uncomplicated pregnancy, during the 3rd trimester after disease onset (described in S2 and S3 Tables). Fifteen of 17 women with preeclampsia delivered pre-term whereas all of the women with gestational hypertension delivered at term. Therefore, women with preeclampsia were matched by gestational age to controls who delivered at term but had provided earlier 3rd trimester samples at the time of routine prenatal care visits. Women with gestational hypertension were matched by gestational age to a different set of controls with samples obtained immediately before delivery.
- Both villous placental tissue and maternal plasma samples were obtained at the time of delivery from 19 women with preeclampsia and 25 women with uncomplicated pregnancies (samples not matched for gestational age at delivery). Clinical characteristics are given in Table 2. Two preeclampsia cases and 2 controls were also in study set #3, above.
- We evaluated soluble Sdc1 levels in plasma from a separate set of 17 women with a history of preeclampsia and 19 with history of uncomplicated pregnancy, 1 year after delivery (S4 Table).
Patient criteria
Women were prospectively recruited in the outpatient clinics or inpatient setting and all deliveries occurred at Magee-Womens Hospital. Patients with a history of chronic hypertension (determined by subject history and/or antihypertensive medication use prior to the pregnancy), diabetes, chronic renal disease or other significant metabolic disorders, current infection, fetal malformations or chromosomal abnormalities, multifetal gestation, or a positive toxicology screen were excluded. A panel of PEPP principal investigators and clinicians met monthly to adjudicate pregnancy outcomes. Controls were normotensive and without proteinuria throughout gestation, and delivered healthy babies in the absence of infection. Preeclampsia was defined as gestational hypertension with proteinuria, in accordance with standard criteria at the time (2000 National High Blood Pressure Education Program Working Group Report on High Blood Pressure in Pregnancy) [31]. Gestational hypertension was defined as persistent, new onset hypertension (systolic ≥ 140 mm Hg and/or diastolic ≥ 90 mm Hg) appearing after 20 weeks’ gestation and reverting post pregnancy. Threshold for proteinuria was ≥ 300 mg of protein in a 24-hour urine collection, a dipstick of 2+ or greater, a catheterized sample of 1+ or greater, or a protein to creatinine ratio ≥ 0.3. Our research-specific criteria for preeclampsia also included gestational hyperuricemia (>1 standard deviation above normal values for gestational age) [32]. The inclusion of hyperuricemia is thought to reduce the number of women misclassified as preeclamptic [32]. Women in the gestational hypertension outcome group manifested hypertension after gestational week 20 but not proteinuria.
Gestational age at delivery was based on last menstrual period and ultrasound, the latter available in most cases. Preterm birth was defined as delivery before 37 completed weeks of pregnancy. Gestational age-specific birth weight percentiles, adjusted for sex and race, were based upon data from Magee-Womens Hospital. Maternal race was by self-report at enrollment. Because of the small percentage of women who identified their race as other than black or white (<3%), results are reported as black or other. Pre-pregnancy body mass index [weight (kg)/height (m)2] was based on measured height and maternal self-report of pre-pregnancy weight at the initial clinic visit. Cigarette smoking status (any smoking since suspected pregnancy) was by self-report; these data were obtained at the time of sample collection; we previously demonstrated no significant discordance between self-report of smoking status and maternal plasma cotinine concentrations [33].
Soluble syndecan-1
Blood samples were collected into sterile, ethylenediaminetetraacetic acid (EDTA)-containing collection tubes. The tubes were centrifuged for 20 min at 2,000 x g at room temperature portioned into aliquots and the plasma stored at −80°C without thaw until used. Concentrations of soluble Sdc1 in maternal plasma were measured in duplicate by ELISA (Diaclone sCD138 solid phase sandwich ELISA, Cell Sciences Inc., Canton, MA.) according to manufacturer’s instructions. The minimum detectable concentration was 4 ng/mL. The intra and inter-assay coefficients of variation were 6 and 10%, respectively. We performed sample dilution tests on separate pools of preeclampsia and uncomplicated pregnancy plasma; there was good linear correlation between the degree of sample dilution and measured Sdc1 concentrations, using 1:4, 1:8, and 1:16 (vol/vol) dilutions of each pool within the dynamic range of the assay (r2 = 0.90).
Placental Immunohistochemical Staining and Scoring
We obtained placental biopsies immediately after elective Cesarean section or vaginal delivery from regions free of infarcts, between the margin and cord insertion. The villous tissue was cut into 0.5 cm3 pieces and rinsed with ice-cold phosphate buffered saline. After rinsing, portions of villous tissue were flash frozen and then placed into embedding medium (optimal cutting temperature compound, OCT, Tissue-Tek, Inc). Frozen OCT-embedded placentas were sectioned (7-μm), fixed in acetone, and peroxidase quenched by 3% H2O2. Acetone-fixed sections are appropriate for surface (CD) proteins [34]. Immunostaining was accomplished using the VECTASTAIN Elite ABC Kit, with anti-Sdc1 ectodomain antibody (H-174 rabbit anti- human polyclonal; sc-5632, Santa Cruz Biotechnology, Dallas TX) at 1:100 dilution, with blocking serum and 3, 3-diaminobenzidine supplied by the kit. Tissues were counterstained with hematoxylin (Vector Laboratories, Burlingame, CA). Negative controls were prepared by replacing the primary antibody with blocking serum. Bright field images were collected using a Nikon90i microscope and CCD camera (QImaging, Surrey, BC, Canada). Visual scoring of the STB of placental villi was performed on 4x magnification images, on a 0–4 semi-quantitative scale with 4 being the darkest stain along the entire apical surface. Images were scored by 2 technicians pre-trained to the scale but unaware of the source of the tissue. The weighted Cohen’s kappa coefficient for the 0–4 scoring between the technicians was 0.64, indicating “substantial” agreement. The values from each scorer were averaged.
Western Blot Comparisons of Syndecan-1 in Placental Homogenates
Details are provided under Supporting Information (S1 Method). Twenty to thirty milligrams of frozen pulverized villous tissue was homogenized by sonication and further prepared for Western blot. Human recombinant CD138 (Diaclone ELISA, Cell Sciences Inc., Canton, MA) was used as a standard, and Precision Plus Protein Kaleidoscope Standards (Bio Rad Technologies, Hercules, CA) were used as molecular weight ladder. Blots were incubated with a rabbit polyclonal antibody against human Sdc1 ectodomain (H-174; Santa Cruz Biotechnology), followed by goat anti-rabbit IgG-HRP (Santa Cruz Biotechnology). Protein bands were detected by enhanced chemiluminescence. We used Amido Black as a loading control, as this total protein stain optimally reflects total protein concentration for semi-quantitative Western blot of placental homogenates [35]. The developed films were scanned into TIFF files and densitometry was carried out using digitizing software, UN-SCANIT™ Gel Version 4.3 (Silk Scientific Inc., Orem, UT).
Isolation and Analysis of Placental RNA
Total cellular RNA was isolated from placental tissue with TRIzol reagent (Invitrogen, Carlsbad, CA) according to the manufacturer instructions. After isolation, double- and single-stranded DNA was removed by treatment with DNase I (Invitrogen). Purity of RNA was determined by measuring absorption at 280 and 260 nm. The cDNA was synthesized from 2 μg of RNA, converted to cDNA using an Applied Biosystems (Foster City, CA) high capacity cDNA kit. Control reactions without reverse transcriptase were performed to confirm the absence of DNA contamination. Quantitative real-time polymerase chain reaction (PCR) was performed on a TaqMan ABI PRISM 7700 sequence detector using 96-well optical plates (Applied Biosystems). Real-time PCR was performed using inventoried TaqMan Gene Expression Assays for syndecan-1 (Hs00896423_m1), syndecan-2 (Hs00299807_m1), glypican-1 (Hs00157805_m1) and 18s (Hs99999901_s1) (Applied Biosystems). The 18s gene expression assay was used as an endogenous reference for all samples. All amplification cycles were performed in a 50μl reaction in duplicate with cDNA equivalent to 100ng of total RNA. Relative quantification of the target expression was performed using the comparative cycle threshold (CT) method as described in Applied Biosystems User Bulletin #2, with normalization of the number of target gene copies (e.g., Sdc1) to an endogenous reference (18s) and relative to a calibrator (an uncomplicated pregnancy placenta).
Statistical Analyses
Continuous data are expressed as median (range). Categorical data are given as frequencies (n) and percentages (%). Longitudinal changes in plasma soluble Sdc1 across normal pregnancy were evaluated using Repeated Measures Analysis of Variance on Ranks with post hoc Student-Newman-Keuls test. Non-parametric tests (Mann-Whitney U or Kruskal-Wallis with post hoc Dunn’s test) or Students’ t-test were used as appropriate to compare continuous data between groups. Between-group placental expression data were compared using ANCOVA on ranks, both with and without adjustment for gestational age. Fisher’s Exact test was used for categorical variables. Spearman’s rho correlations were computed to investigate bivariate relationships.
Results
Maternal plasma soluble Sdc1 concentrations across uncomplicated pregnancy
Clinical characteristics are summarized in S1 Table. As shown in Fig 1, soluble Sdc1 concentrations increased significantly with advancing gestation, with highest values observed just prior to delivery [median ng/ml (range): 1415 (365–3000)], followed by a substantial decrease at 11–17 hours postpartum. With one exception (blood sample obtained 2 days before labor) the pre-delivery samples were obtained after labor onset. In additional samples between 27–29 weeks (before labor) from 4 patients, soluble Sdc1 was nearly double the corresponding value at 17–26 weeks (median mg/mL: 696 vs. 376), further indicative of progressive gestational increases. Soluble Sdc1 at 7–11 weeks of pregnancy [median ng/ml (range): 218 (102–290)] was approximately 7 times higher when compared to 4–9 weeks postpartum [28 (13–114) ng/mL; P<0.05] (Fig 1), the latter not different from values in the women with history of uncomplicated pregnancy assessed 1 year postpartum [27 (13–61) ng/mL; see postpartum data, below.].
Mid-pregnancy comparison of soluble Sdc1
We compared soluble Sdc1 in gestational age-matched, mid-pregnancy (range 18–24 weeks) samples from 9 women who later developed preeclampsia, 9 who developed gestational hypertension (without proteinuria), and 19 with uncomplicated pregnancy. Clinical data are given in Table 1; all were non-smokers. There were no between-group differences in gestational age at the time of blood sampling, maternal age, body mass index, early gestational blood pressures, or racial distribution. All of the gestational hypertensive and uncomplicated pregnancy patients in this cohort delivered at term, with appropriate-for-gestational- age (AGA) infants. As shown in Fig 2, soluble Sdc1 concentrations were significantly lower, weeks before clinical development of preeclampsia [median ng/mL 174 (range 48–353)], but not gestational hypertension [242 (111–1187)], compared to controls [272 (78–1463)] (P<0.05, Kruskal-Wallis, post hoc Dunn’s). Examining the groups separately, we found no significant correlations of soluble Sdc1 with the clinical variables listed in Table 1.
Mid-gestation plasma soluble Sdc1 levels (at median gestational weeks 19.2 vs. 20.1, P = 0.74) were lower in women who later developed early-onset preeclampsia (gestational age at delivery <37 weeks; n = 4) compared to late-onset preeclampsia (gestational age at delivery ≥ 37 weeks; n = 5), but not significantly so [median ng/mL 163 (range 104–207) vs. 195 (158–251); P = 0.38]. The two preeclampsia patients who later delivered SGA infants had mid-gestation soluble Sdc1 values (161 and 217 ng/mL), straddling the overall preeclampsia group median (174 ng/mL).
Cross-sectional 3rd trimester study of soluble Sdc1
We compared 17 women with preeclampsia, with plasma obtained after disease onset, to 17 normotensive controls (S2 Table). The samples were obtained before any labor, and group gestational ages at the time of blood sampling were equivalent. The age, pre-pregnancy body mass index, early gestation blood pressures, percentage of cigarette smokers, and racial distribution did not differ between groups. Circulating soluble Sdc1 was ~2.5-fold lower in women with preeclampsia [median ng/mL (range): preeclampsia 281(101–2237) compared to controls 705 (243–2861); P<0.01]. The median birth weight percentile in the preeclampsia group was 11.3 (range 1–78). Eight of the 17 cases delivered babies that were small-for-gestational-age (SGA), defined as birth weight percentile <10. Median centile of this sub-group was 3 (range 1–9). Despite one high value outlier (2237 ng/mL), soluble Sdc1 levels in this SGA-preeclampsia sub-group were significantly lower than controls [median pg/mL (range): 225 (101–2237); P < 0.01]; these SGA-preeclampsia values, at gestational age 31 (range 26–33) weeks, were comparable to those observed at 7–11 weeks of uncomplicated pregnancy (Fig 1). In contrast, median soluble Sdc1 values in the preeclampsia sub-group with AGA infants [584 (159–1529)] only trended lower than controls (P = 0.18). The gestational age at time of blood sampling did not differ between controls and SGA-preeclampsia or AGA-preeclampsia.
In contrast, among plasma samples matched for gestational age at term prior to delivery (S3 Table), soluble Sdc1 in women with gestational hypertension [median ng/mL (range): 923 (443–1358)] did not differ significantly from controls [1308 (475–1637); P = 0.12].
Soluble Sdc1 correlated with infant birth weight percentile in women with gestational hypertension (r = 0.69, P<0.05) and their controls (r = 0.79, p<0.03), but the correlation was marginal in preeclamptic women (r = 0.45, P = 0.07) and not significant in their controls (r = 0.05, P = 0.84). No other correlations between soluble Sdc1 and clinical variables were found.
Placental Sdc1 expression and plasma soluble Sdc1 in preeclampsia and controls
Preeclampsia and control placentas were evaluated; patient clinical characteristics are summarized in Table 2. Sdc1 immunoreactivity was prominent on villous syncytiotrophoblast but not detected on fetal villous vasculature (Fig 3). There was no discernable between-group difference in localization of the protein. The Sdc1 intensity score for Sdc1 on syncytiotrophoblast was significantly lower in preeclampsia (n = 19) [2.0 (1.0–3.5)] vs. controls (n = 25) [(3.0 (1.0–4.0)] (P<0.001, Unadjusted Rank ANCOVA). This difference remained significant (P<0.02) after adjusting for gestational age (Table 3). Presence or absence of labor did not appear to influence the Sdc1 immunostaining intensity score in either outcome group (data not shown), although the distribution of preeclampsia placenta samples collected in the presence (n = 16) vs. absence (n = 3) of labor was inadequate for meaningful assessment. Comparing the preeclampsia and uncomplicated pregnancy placentas exposed to labor, the intensity score for Sdc1 on syncytiotrophoblast was significantly lower in preeclampsia with labor (n = 16) [2.25 (2.0–3.0)] vs. controls with labor (n = 13) [(3.0 (2.5–4.0)] (P<0.03).
We compared Sdc1 protein expression in villous tissue homogenates by Western blot, using all available samples from the same patients (n = 16 preeclampsia, 16 controls). By densitometry, median Sdc1 band intensities were lower in the preeclampsia group (P< 0.05;Table 3, Fig 3), consistent with the immunohistochemistry scores. However, differences were not significant after adjustment for gestational age (P = 0.13). Sdc1 mRNA expression level in the villous tissue homogenates was roughly half in preeclamptic women (n = 16) compared to controls (n = 20), significant both with and without adjustment for gestational age (Table 3). Syndecan-2 and glypican-1 mRNA did not differ by group.
Soluble Sdc1 was measured in pre-delivery maternal plasma, available from most of these patients (n = 17 preeclampsia, n = 19 controls). Soluble Sdc1 was lower in preeclampsia (P<0.02, Rank ANCOVA), but not after adjustment for gestational age (P = 0.20) (Table 3).
Sdc1 immunostaining score correlated with villous Sdc1 protein (by Western blot) in preeclamptic women (r = 0.52, P<0.05). Plasma soluble Sdc1 levels correlated with both villous Sdc1 protein (r = 0.54, P<0.03) and mRNA (r = 0.59, P<0.02) in controls. Pre-delivery systolic blood pressure correlated inversely (negatively) with immunostaining score in preeclamptic women (r = -0.50, P<0.05), with villous Sdc1 protein in controls (r = -0.75, P<0.001) and with soluble Sdc1 levels in controls (r = -0.54, p<0.03). Although not significant within each group separately, immunostaining score correlated significantly with birth weight percentile (r = 0.43, P<0.01) among all patients combined. Pre-pregnancy BMI correlated inversely with villous Sdc1 protein in both preeclamptic women (r = -0.54, P<0.04) and controls (r = -0.67, P<0.01). Gestational age at delivery correlated significantly with circulating soluble Sdc1 (preeclampsia r = 0.49, P<0.05; control: r = 0.64, P<0.01), and with villous mRNA in controls (r = 0.51, P<0.03).
Soluble Sdc1 concentrations postpartum
We measured plasma soluble Sdc1 one year after completed pregnancy, comparing 17 non-pregnant women with a history of preeclampsia compared to 19 women with a history of uncomplicated pregnancy. These women were studied after first pregnancy, with the exception of two women with history of preeclampsia who were enrolled after their second completed pregnancy (one with recurrent preeclampsia, and one with preeclampsia in second pregnancy but unknown first pregnancy history). Study participants presented to the Magee-Womens Hospital Clinical and Translational Research Center in the morning after an overnight fast. At that time they completed a health history questionnaire, underwent a physical examination (weight, height, blood pressure, heart rate) and a urine test to rule out pregnancy, and a fasting blood sample was obtained. All women were non-lactating at the time, had no pre-pregnancy or current history of renal or vascular disease, and were nonsmokers (S4 Table). Plasma soluble Sdc1 concentrations were not different post-pregnancy [median ng/mL (range): women with a history of preeclampsia 23 (5–72) vs. control 27 (13–61); P = 0.98]. Soluble Sdc1 did not significantly differ post-pregnancy between women with a history of preterm vs. term preeclampsia, or between women with a history of preeclampsia with SGA vs. AGA infants (data not shown). We found no significant correlations of soluble Sdc1 with the clinical variables listed in S4 Table.
Discussion
The glycocalyx is a negatively charged, gel-like mesh on the apical surface of epithelial and endothelial cells. The syncytiotrophoblast microvillous membrane surface has a substantial glycocalyx, but neither its normal functions nor its status/role in pregnancy disorders have been sufficiently evaluated. Both acute insults (e.g., ischemia/reperfusion, volume loading, heart surgery) and chronic conditions (e.g., sepsis, atherosclerosis and diabetes mellitus) can lead to damage to the vascular endothelial glycocalyx[36,37]. Shedding of the Sdc1 extracellular domain, and of other glycocalyx constituents such as hyaluronic acid and heparan sulfate, is normally regulated by matrix metalloproteinases and heparanases. However, shedding can be accentuated by tumor necrosis factor-alpha, oxidized lipoproteins, reactive oxygen species, and other inflammatory mediators [3,10,28,29].
Given evidence for a role of inflammation in the pathogenesis of preeclampsia we originally hypothesized that soluble Sdc1 would be elevated in women with the syndrome. Contrary to hypothesis, we found that soluble Sdc1 concentrations are significantly lower during the second trimester, ~16 weeks before clinically apparent preeclampsia, and in the 3rd trimester after disease onset but before any labor, compared to controls with uncomplicated pregnancy. This pattern in gestational age-matched samples was not observed in women with gestational hypertension compared to controls, suggesting that reduced soluble Sdc1 is not simply a biomarker of hypertensive pregnancy. Although we cannot rule out the presence of subclinical disease at the time of blood sampling, these data suggest that Sdc1 dysregulation is an early event in preeclampsia pathogenesis.
Our study further shows that maternal plasma concentrations of soluble Sdc1 rise progressively with advancing pregnancy. By 7–11 weeks of pregnancy, concentrations were approximately 7-fold higher than values at 4–9 weeks after delivery. Soluble Sdc1 levels at term (median 1415 ng/mL) are similar to those reported during sepsis [10,38], and represent an approximate 50-fold increase over the postpartum levels. Concentrations normalized substantially toward early pregnancy levels within 24–48 hours postpartum. Our data do not preclude the possibility of labor and delivery (uterine contractions) causing a substantial increase in soluble Sdc1; systematic evaluation of this would require serial samples/measurements during the delivery. However, median soluble Sdc1 concentrations in the normotensive controls with 3rd trimester samples obtained before any labor (S2 Table; median gestational week 32.0) were roughly 25-fold higher than values in different controls measured at 4–9 weeks after delivery (Fig 1) or 1 year after delivery.
A prior study reported that serum Sdc1 concentrations rise with normal pregnancy (159-fold increase at term compared to nonpregnancy), with increases occurring mostly between weeks 20 and 30 of pregnancy[39]. However, that study reported even higher serum levels in patients with hemolysis, elevated liver enzymes and low platelets (HELLP) syndrome compared to normotensive controls matched by gestational age. Only two preeclampsia patients in our entire study manifested elevated liver enzymes and thrombocytopenia (hemolysis data not available); soluble Sdc1 values in these patients were below the preeclampsia median. The reason for the discrepancy between the two studies is not clear. However, 40% of the HELLP patients in the cited study did not fulfill diagnostic criteria for preeclampsia, and very few evidently had growth restricted/SGA infants as birth weights were on average 102 grams over that predicted on the basis of customized charts [39]. It is possible that HELLP syndrome as a distinct, severe disease involves widespread glycocalyx shedding with release of soluble glycans.
Using an antibody that recognizes the Sdc1 ectodomain (H-174), we found substantial expression of syndecan-1 on the STB of placental villi, but no detectable expression on fetal villous vasculature. This agrees with previous reports using several different monoclonal or polyclonal anti- Sdc1 antibodies, in which the apical surface of STB was extensively immunoreactive for Sdc1, but not evident on cytotrophoblast, stroma, extravillous trophoblast, or fetal vascular endothelium [21–27]. The rise in soluble Sdc1 with advancing pregnancy combined with the rapid decline in soluble Sdc1 levels within hours after delivery, and the robust expression of Sdc1 on STB in direct contact with maternal blood, suggest that the placenta is a major source of the high levels of soluble Sdc1 in the maternal circulation during pregnancy. Consistent with this hypothesis, we have observed that villous tissue fragments in explant culture produce appreciable amounts of soluble Sdc1 (~10 ng/mg tissue/24 hours) under 8%O2/5%C02, 37°C incubation conditions (author’s unpublished data). However, this does not rule out maternal vasculature or blood cells as a significant source of soluble Sdc1 during pregnancy.
Our immunohistochemical, Western blot and mRNA data collectively indicate a decrease in placental Sdc1 expression with preeclampsia. The lack of matching for gestational age in the evaluation of preeclamptic and control placentas may be viewed as a limitation of our study. Seventeen of 19 preeclamptic placentas but only 2 of 25 controls in our placental analyses delivered preterm (gestational age <37 weeks). Nevertheless, group differences in immunostaining score and mRNA expression were significant after adjustment for gestational age. This agrees with previous reports of reduced expression of Sdc1 on STB microvillous membrane [21,22,25] or decreased Sdc1 in villous homogenates measured by ELISA [25], in which differences from controls were independent of gestational age. There is one contrasting report of an overall increase in expression of Sdc1 on STB, accompanied by significantly lower soluble Sdc1 concentrations in maternal serum, in preeclampsia (with or without HELLP syndrome) compared to normal controls[40]. Using an antibody (MI15) recognizing an extracellular epitope of the core protein, the authors reported focally reduced or absent Sdc1 immunoreactivity on the apical microvillous surface despite an overall increase in cytoplasmic Sdc1 in preeclampsia. When complicated with HELLP syndrome, however, the STB apical surface showed strong Sdc1 positivity. The authors suggested that abnormal cytoplasmic accumulation/retention of Sdc1 below the apical membrane occurs with preeclampsia, and that this might contribute to the observed reduction of soluble Sdc1 in maternal plasma [40]. We similarly used an anti-Sdc1 antibody directed against the extracellular domain but did not have the resolution to distinguish surface from cytoplasmic immunoreactivity. Our Western blot data using villous homogenates show less pronounced group differences in Sdc1 compared to our immunostaining (Table 3), possibly consistent with cytoplasmic versus membrane differences.
Our mid-pregnancy data are consistent with a possible contribution of soluble Sdc1 to the pathogenesis of preeclampsia. It has been hypothesized that both the reduction in circulating placental growth factor (PlGF) and the elevation of the antiangiogenic protein soluble fms-like tryosine kinase 1 [sFLT1; the soluble decoy receptor for PlGF and vascular endothelial growth factor (VEGF)]—that arise before clinically evident preeclampsia—are manifestations of syncytiotrophoblast cellular stress or syncytiotrophoblast dysfunction [41]. Although speculative at this point, the lower soluble Sdc1 in women destined to develop preeclampsia, and evidence of downregulation of syncytiotrophoblast Sdc1 in women with the syndrome, may be another expression of this stress response. The soluble Sdc1 ectodomain is known to function as a paracrine and autocrine effector or competitor, and may have relevant functions by influencing angiogenesis, coagulation pathways, inflammation, or lipid metabolism [14,20,28]. The shed Sdc1 ectodomain can compete with intact syndecan by sequestering heparan sulfate-binding ligands in the pericellular environment [28]. Syndecan-1 shedding is an important host response that facilitates the resolution of neutrophilic inflammation, potentially by aiding the clearance of pro-inflammatory chemokines. Soluble syndecans can act as extracellular chaperones; for example soluble VEGF-Sdc1 complexes can activate VEGF receptors on endothelial cells [14]. An excess of sFLT1 is thought to contribute to the pathogenesis of preeclampsia [42]. Membrane-bound heparan sulfate proteoglycans bind sFLT1 electrostatically, thereby regulating sFLT1 bioavailability [43]. Transgenic heparanase overexpression in mice significantly increases circulating sFLT1 [43]. Concentrations of plasma sFLT1 in non-pregnant women increase to late pregnancy levels shortly after intravenous heparin injection, suggesting that significant quantities of sFLT1 are normally bound to glycocalyx heparan sulfate proteoglycans [44]. However, the influence of membrane-bound or soluble Sdc1 on sFLT1 kinetics and activity has yet to be explored.
Conclusions
Maternal plasma concentrations of shed (soluble) Sdc1 rise ~50-fold with gestation and revert postpartum. On average, women who later develop preeclampsia have lower levels of soluble Sdc1 in maternal plasma at 20 weeks’ gestation (before clinical disease onset) compared to women with uncomplicated pregnancy or gestational hypertension. Differences between women with preeclampsia compared to controls remain evident after disease onset, especially in preeclampsia with SGA neonates. Mirroring the differences in maternal circulation, preeclampsia is characterized by reduced expression Sdc1 on the syncytiotrophoblast of placental villi. There was no difference in concentration of soluble Sdc1 post pregnancy between women with prior preeclampsia and prior uncomplicated pregnancies. These data are collectively consistent with the placenta as a major source of soluble Sdc1, likely shed into the circulation at reduced quantity in women who develop preeclampsia. The study datasets were small and should be validated with larger cohorts. Because of the observational nature of our study we cannot establish a causal relationship between maternal plasma Sdc1 levels and the subsequent development of preeclampsia. Further studies are needed to determine the effects of altered Sdc1 kinetics on pregnancy physiology and its significance for placental and vascular glycocalyx integrity and function in normal pregnancies and those complicated by preeclampsia.
Supporting Information
S1 Data. Longitudinal Syndecan.
(XLSX)
S2 Data. 20 week Syndecan.
(XLSX)
S3 Data. Third Trimester Syndecan.
(XLSX)
S4 Data. Placenta and Syndecan.
(XLSX)
S1 Method. Western blots of Placental Homogenate.
(DOCX)
S1 Table. Clinical characteristics of uncomplicated pregnancy patients (n = 8), for measurement of soluble Sdc1 concentration in maternal plasma samples collected longitudinally during and after pregnancy. Continuous variables are given as median (range); categorical variables are displayed as n (%).
(DOCX)
S2 Table. Clinical characteristics of uncomplicated pregnancy and preeclampsia groups for evaluation of soluble Sdc1 concentration in gestational age-matched 3rd trimester maternal plasma samples. Continuous variables are displayed as median (range); categorical variables displayed as n (%). N/M: not measured. a Data missing for 1 woman with uncomplicated pregnancy and 5 women with preeclampsia.
(DOCX)
S3 Table. Clinical characteristics of uncomplicated pregnancy and gestational hypertension groups for evaluation of soluble Sdc1 concentration in gestational age-matched 3rd trimester maternal plasma samples. Continuous variables are displayed as median (range); categorical variables displayed as n (%). a Data missing for 1 woman with uncomplicated pregnancy.
(DOCX)
S4 Table. Clinical characteristics; comparison study of plasma soluble Sdc1 concentrations 1 year after pregnancy. Continuous variables are given as median (range); categorical variables displayed as n(%).
(DOCX)
Acknowledgments
We thank the Clinical Data Core and PEPP study staff of Magee-Womens Hospital for invaluable assistance.
Author Contributions
Conceived and designed the experiments: CAH REG. Performed the experiments: SM ACM MG AJ RWP. Analyzed the data: AA REG JMB-W CAH. Contributed reagents/materials/analysis tools: CAH REG. Wrote the paper: CAH REG RWP AJ JMB-W.
Competing interests
The authors have declared that no competing interests exist.
Funding
This project was supported by the State of Pennsylvania Health Research Formula Fund (CAH)(portal.state.pa.us), and National Institutes of Health Grants R21HD083659 (REG and CAH), P01HD030367 (CAH, REG, AJ, SM, ACM,RWP), and UL1RR024153 and UL1TR000005 (University of Pittsburgh Clinical and Translational Science Institute)(nih.gov). The funders had no role in study design, data collection and analysis, decision to publish, or preparation of the manuscript.
Data Availability
The data contained in this manuscript includes protected health information and will therefore be made available to all interested researchers upon request. Abbreviated datasets have been made available with the manuscript. Requests for access to additional data should be directed to Dr Gandley or Hubel, or the PEPP executive committee at the Magee Womens Research Institute and is subject to the approval of the University of Pittsburgh Human Research Protection Office, 3500 5th Ave. Hieber Building Suite 106, Pittsburgh PA 15213, Phone 412-383-1480, email: irb@pitt.edu.
Editor: Ana Claudia Zenclussen, Medical Faculty, Otto-von-Guericke University Magdeburg, Medical Faculty, GERMANY
Received: March 14, 2016
Accepted: June 1, 2016
Published: June 14, 2016
A. Hubel
Department of Obstetrics, Gynecology & Reproductive Sciences
Division of Maternal Fetal Medicine, University of Pittsburgh
Pittsburgh, Pennsylvania, United States of America
chubel@mwri.magee.edu
Zdroje
1. Jansson T, Myatt L, Powell TL. The role of trophoblast nutrient and ion transporters in the development of pregnancy complications and adult disease. Curr Vasc Pharmacol. 2009; 7(4):521–33. PMID: 19485888
2. Burton GJ, Woods AW, Jauniaux E, Kingdom JC. Rheological and physiological consequences of conversion of the maternal spiral arteries for uteroplacental blood flow during human pregnancy. Placenta. 2009; 30(6):473–82. PMID: 19375795 doi: 10.1016/j.placenta.2009.02.009
3. Tarbell JM, Cancel LM. The glycocalyx and its significance in human medicine. J Intern Med. 2016 Jan 8: doi: 10.1111/joim.12465 PMID: 26749537
4. Singh A, Satchell SC, Neal CR, McKenzie EA, Tooke JE, Mathieson PW. Glomerular endothelial glycocalyx constitutes a barrier to protein permeability. J Am Soc Nephrol. 2007; 18:2885–93. PMID: 17942961
5. Becker BF, Chappell D, Jacob M. Endothelial glycocalyx and coronary vascular permeability: the fringe benefit. Basic Res Cardiol. 2010; 105:687–701. PMID: 20859744 doi: 10.1007/s00395-010-0118-z
6. Broekhuizen LN, Lemkes BA, Mooij HL, Meuwese MC, Verberne H, Holleman F, et al. Effect of sulodexide on endothelial glycocalyx and vascular permeability in patients with type 2 diabetes mellitus. Diabetologia. 2010; 53:2646–55. PMID: 20865240 doi: 10.1007/s00125-010-1910-x
7. Annecke T, Fischer J, Hartmann H, Tschoep J, Rehm M, Conzen P, et al. Shedding of the coronary endothelial glycocalyx: effects of hypoxia/reoxygenation vs ischaemia/reperfusion. British Journal of Anaesthesia. 2011; 107:679–86. PMID: 21890663 doi: 10.1093/bja/aer269
8. Nussbaum C, Cavalcanti F, Heringa A, Mormanova Z, Puchwein-Schwepcke AF, Bechtold-Dalla Pozza S, et al. Early microvascular changes with loss of the glycocalyx in children with type 1 diabetes. J Pediatr. 2014; 164:584–9 e1. PMID: 24367980 doi: 10.1016/j.jpeds.2013.11.016
9. Lemkes BA, Nieuwdorp M, Hoekstra JB, Holleman F. The glycocalyx and cardiovascular disease in diabetes: should we judge the endothelium by its cover? Diabetes Technol Ther. 2012; 14 Suppl 1:S3–10. PMID: 22650222 doi: 10.1089/dia.2012.0011
10. Steppan J, Hofer S, Funke B, Brenner T, Henrich M, Martin E, et al. Sepsis and major abdominal surgery lead to flaking of the endothelial glycocalix. J Surg Res. 2011; 165:136–41. PMID: 19560161 doi: 10.1016/j.jss.2009.04.034
11. Bradbury S, Billington WD, Kirby DR, Williams EA. Histochemical characterization of the surface mucoprotein of normal and abnormal human trophoblast. Histochem J. 1970; 2:263–74. PMID: 4260517
12. Nelson DM, Smith CH, Enders AC, Donohue TM. The nonuniform distribution of acidic components on the human placental syncytial trophoblast surface membrane: a cytochemical and analytical study. Anat Rec. 1976; 184:159–81. PMID: 1247183
13. Hofmann-Kiefer KF, Chappell D, Knabl J, Frank HG, Martinoff N, Conzen P, et al. Placental syncytiotrophoblast maintains a specific type of glycocalyx at the fetomaternal border: the glycocalyx at the fetomaternal interface in healthy women and patients with HELLP syndrome. Reprod Sci. 2013; 20:1237–45. PMID: 23585336 doi: 10.1177/1933719113483011
14. Teng YH, Aquino RS, Park PW. Molecular functions of syndecan-1 in disease. Matrix Biol. 2012; 31:3–16. PMID: 22033227 doi: 10.1016/j.matbio.2011.10.001
15. Stepp MA, Pal-Ghosh S, Tadvalkar G, Pajoohesh-Ganji A. Syndecan-1 and its expanding list of contacts. Adv Wound Care (New Rochelle). 2015; 4:235–49. PMID: 25945286
16. Lamorte S, Ferrero S, Aschero S, Monitillo L, Bussolati B, Omede P, et al. Syndecan-1 promotes the angiogenic phenotype of multiple myeloma endothelial cells. Leukemia. 2012; 26:1081–90. PMID: 22024722 doi: 10.1038/leu.2011.290
17. Stanford KI, Bishop JR, Foley EM, Gonzales JC, Niesman IR, Witztum JL, et al. Syndecan-1 is the primary heparan sulfate proteoglycan mediating hepatic clearance of triglyceride-rich lipoproteins in mice. The Journal of Clinical Investigation. 2009; 119:3236–45. PMID: 19805913 doi: 10.1172/JCI38251
18. Tkachenko E, Rhodes JM, Simons M. Syndecans: new kids on the signaling block. Circ Res. 2005; 96:488–500. PMID: 15774861
19. Deng Y, Foley EM, Gonzales JC, Gordts PL, Li Y, Esko JD. Shedding of syndecan-1 from human hepatocytes alters very low density lipoprotein clearance. Hepatology. 2012; 55:277–86. PMID: 21898481 doi: 10.1002/hep.24626
20. Leonova EI, Galzitskaya OV. Lipids in Protein Misfolding. In: Advances in Experimental Medicine and Biology. Switzerland. Springer International Publishing; 2015. p. 241–58.
21. Jokimaa V, Inki P, Kujari H, Hirvonen O, Ekholm E, Anttila L. Expression of syndecan-1 in human placenta and decidua. Placenta. 1998; 19:157–63. PMID: 9548182
22. Jokimaa VI, Kujari HP, Ekholm EM, Inki PL, Anttila L. Placental expression of syndecan 1 is diminished in preeclampsia. American Journal of Obstetrics & Gynecology. 2000; 183:1495–8. PMID: 11120517
23. Crescimanno C, Marzioni D, Paradinas FJ, Schrurs B, Muhlhauser J, Todros T, et al. Expression pattern alterations of syndecans and glypican-1 in normal and pathological trophoblast. J Pathol. 1999; 189:600–8. PMID: 10629564
24. Chui A, Murthi P, Brennecke SP, Ignjatovic V, Monagle PT, Said JM. The expression of placental proteoglycans in pre-eclampsia. Gynecol Obstet Invest. 2012; 73:277–84. PMID: 22516801 doi: 10.1159/000333262
25. Heyer-Chauhan N, Ovbude IJ, Hills AA, Sullivan MH, Hills FA. Placental syndecan-1 and sulphated glycosaminoglycans are decreased in preeclampsia. J Perinat Med. 2014; 42: 329–38. PMID: 24222257 doi: 10.1515/jpm-2013-0097
26. Chui A, Zainuddin N, Rajaraman G, Murthi P, Brennecke SP, Ignjatovic V, et al. Placental syndecan expression is altered in human idiopathic fetal growth restriction. American Journal of Pathology. 2012; 180:693–702. PMID: 22138583 doi: 10.1016/j.ajpath.2011.10.023
27. Gunatillake T, Chui A, Said JM. The role of placental glycosaminoglycans in the prevention of preeclampsia. J Glycobiol. 2013 Feb; 2(1):105. doi: 10.4172/2168-958X.1000105
28. Manon-Jensen T, Multhaupt HA, Couchman JR. Mapping of matrix metalloproteinase cleavage sites on syndecan-1 and syndecan-4 ectodomains. The FEBS journal. 2013; 280:2320–31. PMID: 23384311 doi: 10.1111/febs.12174
29. Burke-Gaffney A, Evans TW. Lest we forget the endothelial glycocalyx in sepsis. Critical Care. 2012 April; 16(121): p. 1–2. doi: 10.1186/cc11239 PMID: 22494667
30. Redman CW, Sacks GP, Sargent IL. Preeclampsia: An excessive maternal inflammatory response to pregnancy. American Journal of Obstetrics & Gynecology. 1999; 180(2 Part 1):499–506. PMID: 9988826
31. Report of the National High Blood Pressure Education Program Working Group on High Blood Pressure in Pregnancy. American Journal of Obstetrics & Gynecology. 2000; 183:S1–S22. PMID: 10920346
32. Chesley LC. Hypertension in pregnancy: Definitions, familial factor, and remote prognosis. Kidney Int. 1980; 18:234–40. PMID: 7003201
33. Jeyabalan A, Powers RW, Durica AR, Harger GF, Roberts JM, Ness RB. Cigarette smoke exposure and angiogenic factors in pregnancy and preeclampsia. Am J Hypertens. 2008; 21:943–7. PMID: 18566591 doi: 10.1038/ajh.2008.219
34. Shi SR, Liu C, Pootrakul L, Tang L, Young A, Chen R, et al. Evaluation of the value of frozen tissue section used as "gold standard" for immunohistochemistry. Am J Clin Pathol. 2008; 129:358–66. PMID: 18285257 doi: 10.1309/7CXUYXT23E5AL8KQ
35. Lanoix D, St-Pierre J, Lacasse AA, Viau M, Lafond J, Vaillancourt E. Stability of reference proteins in human placenta: general protein stains are the benchmark. Placenta. 2012; 33:151–6. PMID: 22244735 doi: 10.1016/j.placenta.2011.12.008
36. Maksimenko AV, Turashev AD. No-reflow phenomenon and endothelial glycocalyx of microcirculation. Biochem Res Int. 2012 September 20; 2012(859231): p.1–10. PMID: 22191033
37. Becker BF, Jacob M, Leipert S, Salmon AH, Chappell D. Degradation of the endothelial glycocalyx in clinical settings: searching for the sheddases. Br J Clin Pharmacol. 2015; 80:389–402. PMID: 25778676 doi: 10.1111/bcp.12629
38. Sallisalmi M, Tenhunen J, Yang R, Oksala N, Pettila V. Vascular adhesion protein-1 and syndecan-1 in septic shock. Acta Anaesthesiol Scand. 2012; 56:316–22. PMID: 22150439 doi: 10.1111/j.1399-6576.2011.02578.x
39. Hofmann-Kiefer KF, Knabl J, Martinoff N, Schiessl B, Conzen P, Rehm M, et al. Increased serum concentrations of circulating glycocalyx components in HELLP syndrome compared to healthy pregnancy: an observational study. Reprod Sci. 2013; 20:318–25. PMID: 22872545 doi: 10.1177/1933719112453508
40. Szabo S, Xu Y, Romero R, Fule T, Karaszi K, Bhatti G, et al. Changes of placental syndecan-1 expression in preeclampsia and HELLP syndrome. Virchows Arch. 2013; 463:445–58. PMID: 23807541 doi: 10.1007/s00428-013-1426-0
41. Redman CWG, Staff AC. Preeclampsia, biomarkers, syncytiotrophoblast stress, and placental capacity. Am J Obstet Gynecol. 2015; 213(4 Suppl):S9.e1, S9-11. doi: 10.1016/j.ajog.2015.08.003
42. Maynard SE, Venkatesha S, Thadhani R, Karumanchi SA. Soluble Fms-like tyrosine kinase 1 and endothelial dysfunction in the pathogenesis of preeclampsia. Pediatr Res. 2005; 57(5 Pt 2):1R–7R. PMID: 15817508
43. Sela S, Natanson-Yaron S, Zcharia E, Vlodavsky I, Yagel S, Keshet E. Local retention versus systemic release of soluble VEGF receptor-1 are mediated by heparin-binding and regulated by heparanase. Circ Res. 2011; 108:1063–70. PMID: 21415391 doi: 10.1161/CIRCRESAHA.110.239665
44. Weissgerber TL, Rajakumar A, Myerski AC, Edmunds LR, Powers RW, Roberts JM, et al. Vascular pool of releasable soluble VEGF receptor-1 (sFLT1) in women with previous preeclampsia and uncomplicated pregnancy. J Clin Endocrinol Metab. 2014; 99:978–87. PMID: 24423299 doi: 10.1210/jc.2013-3277
Článok vyšiel v časopise
PLOS One
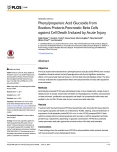
2016 Číslo 6
- Metamizol jako analgetikum první volby: kdy, pro koho, jak a proč?
- Očkování proti virové hemoragické horečce Ebola experimentální vakcínou rVSVDG-ZEBOV-GP
- Nejasný stín na plicích – kazuistika
- Ne každé mimoděložní těhotenství musí končit salpingektomií
- Úspěšná resuscitativní thorakotomie v přednemocniční neodkladné péči
Najčítanejšie v tomto čísle
- Low Soluble Syndecan-1 Precedes Preeclampsia
- Phenylpropenoic Acid Glucoside from Rooibos Protects Pancreatic Beta Cells against Cell Death Induced by Acute Injury